Bumi Gemilang
Blog Pendidikan dan Informasi

SPM Physics Notes (Form 4, 5) : Modifications And Explanations
- SPM Physics Notes (Form 4, 5) : Modifications And Explanations (21)
Sijil Pelajaran Malaysia. Calon SPM wajib menduduki semua kertas peperiksaan bagi mata pelajaran yang didaftar.
Calon yang tidak dapat menduduki mana-mana kertas peperiksaan bertulis atau melaksanakan ujian lisan khusus calon persendirian akan dianggap TIDAK HADIR bagi mata pelajaran berkenaan. Calon yang tidak dapat menduduki atau tidak hadir sesuatu kertas peperiksaan dikehendaki mengemukakan dokumen sokongan seperti surat perakuan doktor atau surat kebenaran khas Pengarah Peperiksaan.
……………………………………………………………………………………..
…………………………………………………………………………………………
- KOLEKSI Soalan Peperiksaan Percubaan SPM + Skema Jawapan (Semua Subjek) (Terkini)
- KOLEKSI Soalan Peperiksaan Percubaan PT3 + Skema Jawapan (Semua Subjek) (Terkini)
- KOLEKSI Soalan Peperiksaan Percubaan Tahun 6 + Skema Jawapan (Semua Subjek) (Terkini)
……………………………………………………………………………………………
- Koleksi Nota Ringkas dan Padat, Soalan, Latihan, KBAT, Peperiksaan, Ujian Tingkatan 1, 2, 3, 4, 5 dan Tahun 1, 2, 3, 4, 5, 6
…………………………………………………………………………………………….
- Bahan-Bahan Rujukan Penting dan Berguna untuk Subjek-Subjek Utama S PM, PT3, Tingkatan 1, 2, 3, 4, 5, d an Tahun 1, 2, 3, 4, 5, 6
- Bahan-Bahan Rujukan Penting: PT3, SPM, STPM, MUET, Tahun 1, 2, 3, 4, 5, 6, dan Tingkatan 1, 2, 3, 4, 5, 6
Untuk mendapatkan segala Maklumat Terkini atau Updates blog Bumi Gemilang .
(1) Follow / Like Bumi Gemilang Facebook Page .
- https://www.facebook.com/BumiGemilang2
(2) Join / Sertai 2 Telegram Groups blog Bumi Gemilang .
- Join / Sertai 2 Telegram Groups To Follow Blog Bumi Gemilang
(3) Follow Twitter blog Bumi Gemilang .
- https://twitter.com/BumiGemilang
………………………………………………………………………………….
Please Share This Post :
Write a comment cancel reply.
Academia.edu no longer supports Internet Explorer.
To browse Academia.edu and the wider internet faster and more securely, please take a few seconds to upgrade your browser .
Enter the email address you signed up with and we'll email you a reset link.
- We're Hiring!
- Help Center
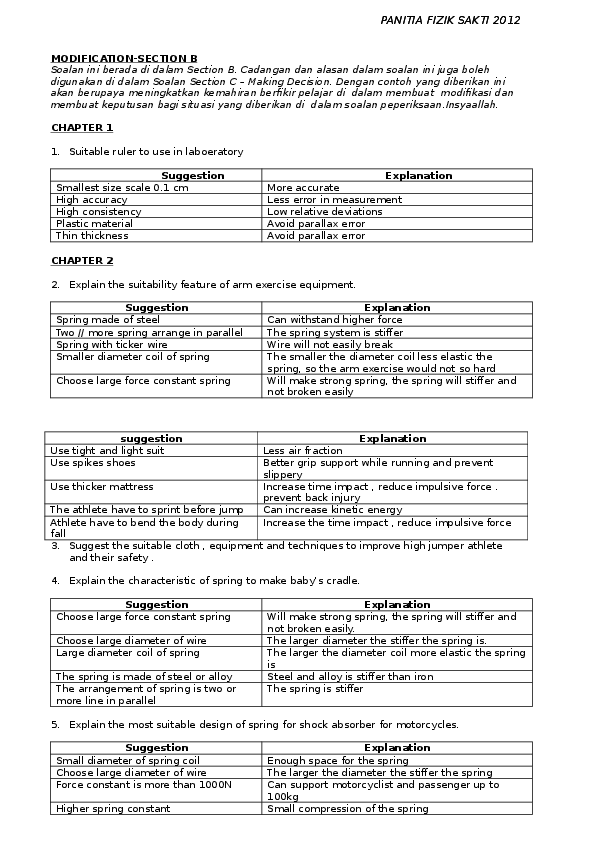
Modification all new physic spm

Related Papers
CHALINE _04
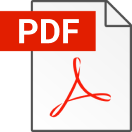
Wen You Chan
Essay questions Problem solving and decision making
Ahmad Nadzhan
tareq islam
fizik smkbidor
sudhir chopra
achmad riyadi
Pablo Fuente
tara radeffy
Loading Preview
Sorry, preview is currently unavailable. You can download the paper by clicking the button above.
RELATED PAPERS
Ammar Aadil
Del Piero Flores
Andres Ruiz Sosa
Muugii Munerd
Physics Grade 10-12 for Highschool
Joaquín Villatoro Alcaraz
Trai Unyapoti
ISXAQ OSMAN ABDIRAHMAN
basit jawwad
Fahad Iftikhar
Samson Ajibade
Nantiporn Tareram
abraham john
Abdullah Majid
Pokhara school
Anh Thư Ngô
Muhammad Habibie
New theories on electromagnetism and on light and colors
Mitko Gorgiev
sanjay taylor
Manuel Docog
YASSON TWINOMUJUNI
- We're Hiring!
- Help Center
- Find new research papers in:
- Health Sciences
- Earth Sciences
- Cognitive Science
- Mathematics
- Computer Science
- Academia ©2024
- No category
physics spm essay example 2
Related documents.
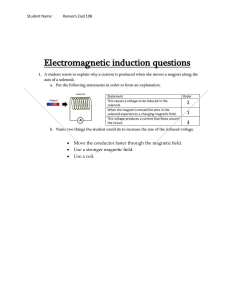
Add this document to collection(s)
You can add this document to your study collection(s)
Add this document to saved
You can add this document to your saved list
Reviews of Modern Physics
- Editorial Team
Feedback for physicists: A tutorial essay on control
John bechhoefer, rev. mod. phys. 77 , 783 – published 31 august 2005.
- Citing Articles (227)
- Introduction
- Brief Review of Dynamical Systems
- Feedback: An Elementary Introduction
- Feedback and Stability
- Implementation and Some Advanced Topics
- Notes on Nonlinearity
- Applications to Biological Systems
- Other Applications, Other Approaches
- Feedback and Information Theory
- Conclusions
- ACKNOWLEDGEMENTS
Feedback and control theory are important ideas that should form part of the education of a physicist but rarely do. This tutorial essay aims to give enough of the formal elements of control theory to satisfy the experimentalist designing or running a typical physics experiment and enough to satisfy the theorist wishing to understand its broader intellectual context. The level is generally simple, although more advanced methods are also introduced. Several types of applications are discussed, as the practical uses of feedback extend far beyond the simple regulation problems where it is most often employed. Sketches are then provided of some of the broader implications and applications of control theory, especially in biology, which are topics of active research.
DOI: https://doi.org/10.1103/RevModPhys.77.783
©2005 American Physical Society
Authors & Affiliations
- Department of Physics, Simon Fraser University, Burnaby, British Columbia V5A 1S6, Canada
- * Electronic address: [email protected]
Article Text (Subscription Required)
References (subscription required).
Vol. 77, Iss. 3 — July - September 2005
Access Options
- Buy Article »
- Log in with individual APS Journal Account »
- Log in with a username/password provided by your institution »
- Get access through a U.S. public or high school library »
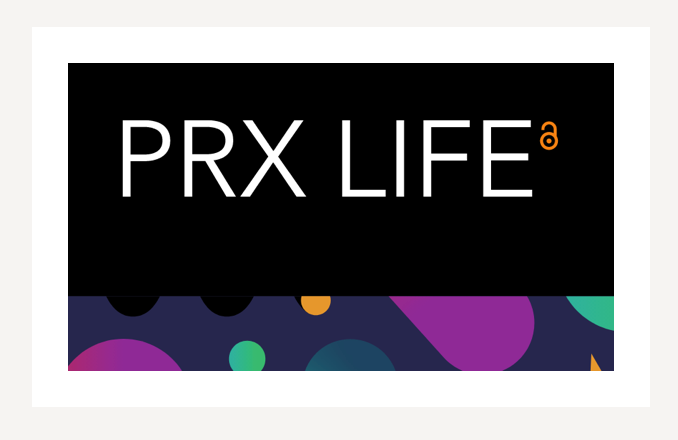
Authorization Required
Other options.
- Buy Article »
- Find an Institution with the Article »
Download & Share
Sign up to receive regular email alerts from Reviews of Modern Physics
- Forgot your username/password?
- Create an account
Article Lookup
Paste a citation or doi, enter a citation.
- Quick Upload
- Contact Us
- FAQ
- Help Document
- Help Document
TIPS PHYSICS SPM 2020
- Cikgu Rizal MRSM AG
- http://anyflip.com/iyki/nexi/
Related Publications
Answering Question Techniques Physics SPM (4531) Paper 1 (50 marks) Time: 1 1/4 hours 50 Multiple Choices Paper 2 (100 marks) Time: 2 1/2 hours 3 Sections Section A: Structural Questions Section B: Restricted Respond & Extended/Open Respond Questions Section C : Restricted Respond & Extended/Open Respond Questions Format of Question Section A : 60% Answer All Questions (7-8 Questions) Section B : 20% (Two questions choose one) (Application & Problem Solving) Section C : 20% (Two questions choose One) (Conceptualisation & Making Decision) Paper 3 (40 marks) Time: 1 1/2 hours 2 Sections Paper 3: Section A – 28 % Consists of 2 questions. Answer all questions. Answer MUST with unit. Question based on experiment that can carry out in the school laboratory. Question 1: Directly proportional Increases linearly Decreases linearly Question 2: tion of graph Eye must perpendicularly to the reading of ( ) to avoid parallax error (example) Paper 3: Section B – 12 % Consists of 2 questions. Answer only 1 question. Full mark is 13/12. Given a diagram of a situation in our daily lives with a brief write-up on the situation. Find the variables (MV & RV) in the situation given (diagram & brief write-up in question). Can give different answer for same question. State an inference depends on MV influenced by MV affected by MV State a hypothesis. When MV increases, RV increases/decreases. The more/higher the MV, the more/higher/lower the RV. Describe an experiment to investigate the hypothesis stated with use of suggested apparatus in the question. To investigate (study) the relationship between MV and RV. MV, RV & CV* (CV can answer whatever possible physical quantities.) pparatus and materials. MUST suggest: metre rule. instrument to measure MV&RV must have) gh time to write) must label. functional arrangement of the apparatus. variable. 4 times. Table of 2 columns (MV & RV) 2 axis only (MV & RV) without any line of graph Can describe more than one experiment for a same question. CHARACTERISTICS OR PROPERTIES MARKING SCHEME FOR MODIFICATIONS DAN MAKING DICISIONS QUESTIONS Chapter 1/2/3 (FORCES AND MOTION/PRESSURE) NO ASPECT/CHARACTERISTIC/MODIFICATION REASON/EXPLANATION 1 The small reading of scale 0.01 cm More sensitif/ accurate/suitable to measure a small length 2 Front and rear crumple zones To increase time of impact //To reduce impulsive force. 3 Air bags Will inflate during collision/to prevent driver and passenger colliding with steering wheel and dashboard. 4 Dashboard - made of soft material To lengthen the time of impact so as to reduce impulsive force. 5 Seat belt To prevent passengers thrown foward due to it inertia 6 Headrest To prevent head thrown back due to its inertia 7 Thicker wall at the base To withstand greater pressure at the bottom as the pressure increases with depth 8 The wall is constructed using stronger To avoid the wall from breaking //To increase the materials //Using reinforce concrete strength of the wall //To avoid leaking To avoid flooding //To channel away the overflow 9 Equipped with the water overflow system water So that the vehicles becomes more stable 10 The mass must be high So the cost is low 11 The types of engine is diesel So the pressure is low // more stable 12 The diameter of the tyre must be bigger Reduce the resistance of water 13 Streamline Higher buoyant force 14 Low Density Absorbs heat slowly 15 Specific heat capacity high Difficult to damage 16 High strength Glass does not corrode with acid 17 Material made from glass To increase the sensitivity of the hydrometer 18 Small diameter of capillary tube Makes the hydrometer stays upright//Lower center of 19 High density of shots/added more gravity To obtain a bigger upthrust/stability 20 Big diameter of bottom bulb Reducing of air friction 21 Aerodynamics //Cone shape at top Higher rate of acceleration//Easy to carry 22 Small mass Enough space for increasing air pressure//Big buoyant 23 Less than half of the bottle filled with force The stability of the rocket water(water roket) Increase the flight distance 24 3 or 4 wings So that it is lighter//Accelerate faster 25 Angle of projection = 45 degree Bigger inertia/stability 26 Low density of an object To produce high acceleration//High resultant force 27 Higher density So that the spring is stiffer //Motorcycle bounce less 28 High engine power //Less vibration 29 High spring constant //Stiffer spring To increase stability//To reduce friction 30 Wide tyre //Smooth tyre So that k is bigger 31 Use a spring with a bigger diameter the type of material influences k produces a bigger 32 The spring is made from steel elastic PE. Elastic PE. changes to KE. a larger k(spring constant) So that elastic Potential Energy is bigger 33 Spring is greatly compressed So that distance is maximum 34 Slope of inclined plane is 45 degrees To be able to withstand high temperature 35 The melting point should be high 36 The material must be very strong To be able to withstand very strong force 37 Shaped with a curved surface at the top To achieve an upward lifting force when moving at high and a flat surface at the bottom speed (aerodynamic) 38 Run with higher speed To increase kinetic energy 39 Bend pole greater //Jump when the pole is Increase elastic potential energy maximum bend 40 Elastic pole // strong material // low So that the pole can return to it original shape // So density that it will not break // light 41 Wear fit attire Reduce air resistance 42 Use mattress/soft material Increase time of collision // reduce impulsive force//increase landing time 43 Made of concrete Stronger // Not easy to break // metal can rust easily 44 Thicker wall at the bottom Able to withstand the higher pressure at the bottom 45 Height from ground is high (dam) To produce a greater difference in pressure 46 Wide base cross section area So that ship can float//prevent from overturn // ship more stable // ship not sink deeper 47 High volume of air space in the ship Produce bigger buoyant force// ship can float 48 Spring arrange in parallel The spring sistem is stiffer/less extension/less elastic 49 Spring with thicker wire The spring is stiffer/wire not easily break 50 Spring with smaller diameter of coil Increase the stiffness of the spring//can with stand higher force 51 Rope with small diameter (parachute) Accupy less space/less mass 52 Long stem(for hydrometer) Cover a wider range of densities 53 Stem with smaller diameter Sink more and increase the sensitivity 54 Low rate of rusting To ensure tha material last longer 55 Semicircular curve shaped(for slope) Exchange between KE and GPE easily 56 Smooth surface Easily to move/reduce frictional force 57 Synthetic material Light weight/air-proof material Chapter 4 (HEAT) NO ASPECT/CHARACTERISTIC/MODIFICATION REASON/EXPLANATION 1 The lid of the pan designed to lower the The boiling point of water decreased air pressure inside the pan 2 The lid of the pan made of substance which Heat will not absorbed by the the lid, so heat will not has weak conductivity of heat lost to surrounding 3 Made from material with low specific heat Temperature in the pot can be increased quickly when capacity heated. This saves fuel / cooking gas. 4 Made from a low density material Pot is light and more portable 5 Made from material that is not easily Pot is more durable and will not contaminate the food corroded or oxidized with dangerous material 6 The handle of the pot is made from The handle becomes hot slower and can be held material with high specific heat capacity without scorching the hand 7 The pot is designed to have vertical This makes the pot versatile because different food can compartments which can be added or be cooked at the same time removed 8 High melting point Does not melt easily if there is an increase in temperature. 9 Liquid that difficult to compress. Pressure will be transmited uniformly in all directions/ flows easily 10 High degree of hardness Can withstand great force // does not break easily 11 Large numbers of fin blade (Engines) Increase surface area // release heat quickly // engine cools quickly 12 Big size of fan Can suck more air // more air can be blow to the engine /Can cool down a larger area 13 High boiling point Not easily to vaporize // the volume of liquid reduce slowly // takes a longer time to boil 14 High specific heat capacity Takes a longer time to become hot // the rise in temperature is slow 15 Increase the length/area of cooling coil Increase the resistance/Can transfer the heat faster to the surrounding 16 A storage tank must be place at a higher To give higher pressure level 17 Pipe embedded in plate must be long Will enlarge surface area will absorbs heat faster 18 The pipe inside the plate must be made of Metal is a good heat conductor,so it will transmit heat metal to water easily 19 Thermometer is made from strong Not easily broken transparent glass 20 Thermometric liquid chosen is mercury Because it easily expands uniformly 21 Capillary tube is made narrow and thin More sensitive 22 Shape of the thermometer is round Has magnifying effect 23 Thermometer is placed in melting ice To obtain the lower point 24 Thermometer is placed in steam To obtain the upper point 25 Low specific heat capacity of ice cream box Easy get cold // becomes cool quickly 26 Smaller size of ice cream box Easier to carry // easy too become cool 27 Plastic PVC Poor conductor of heat 28 Bright colour of outer box Does not absorb heat from surrounding quickly 29 Use insulator behind the absorber panel To prevent the loss of heat energy 30 Use an absorber panel which is painted A black surface is a good absorber of radiation so it will black. absorb heat faster Chapter 5 (LIGHT) NO ASPECT/CHARACTERISTIC/MODIFICATION REASON/EXPLANATION 1 Small critical angle. Allow more light to involve in total internal reflection 2 Strong material Not easily broken. 3 Flexible material. Can easily change the shape. 4 Fine diameter Can enter small holes. 5 High refractive index Total internal reflection can occur easily 6 Optical fibre in a bundle Large number of signal/higher intensity of light can propagate 7 Material with weak rigidity The optical fibre can be bent easily 8 Material with great strength The optical fibre can last longer//not easily spoil 9 A plane mirror mounted on an adjustable Reflects light to the vertical screen, corrects lateral and arm vertical inversion 10 Use a converging mirror instead of plane Focus the light directly to the lens // increase the mirror intensity of light. 11 Place the filament at the centre of Light goes directly from the lamp and reflect back on curvature of the converging mirror // use the same path // increase the intensity of light towards high powered lens the transparency // to get brighter image 12 Use heat filter To absorb excess heat to the transparency 13 Electric fan operates during and after the Cooling system to stabilize the temperature (heat lamp is switched on energy produced by filament bulb) 14 Shorter // smaller size of binocular easy to carry 15 Use prism to make the total internal Produce upright image reflection occur 16 Higher density Higher refractive indeks 17 Objective lens with larger diameter More light passes through the lens 18 Eyepiece with higher power Shorter focal length 19 Shorter focal length Higher power/increase the magnification 20 Convex lens Can produce real image 21 u a bit bigger than f Produce maximum magnification 22 Periscope Cheaper than CCTV 23 2 plane mirror/prism Can reflect light from object 24 Casing to hold the mirror Easier to handle periscope 25 Convex mirror Wider field view 26 Optical fibre with higher densities/ To ensure total internal reflection occur refractive index inner compare than outer Form 5 Chapter 6 (WAVE) NO ASPECT/CHARACTERISTIC/MODIFICATION REASON/EXPLANATION 1 Build near bay Waves are calmer due to divergence of energy// Convergence of waves at the cape//The bay is 2 Build retaining walls shallower//The speed of waves decreases//The amplitude of waves at the bay is small 3 Concrete barrier structure with a gap in Reduce direct impact of the waves on the shore. To between reflect the waves from the shore//Protect the area from large waves //Avoid erosion 4 Build high retaining wall Waves passing through the gap will be diffracted in the 5 Thick area at the base of the wall children’s area/the smaller amplitude of the diffracted 6 Long wavelength waves causes the sea to be calmer there energy of 7 Short Slit waves decreases. 8 Ultrasonic wave To ensure the water not overflow. To withstand high pressure at the base Easy to diffract Diffraction more obvious Can transfer more energy Chapter 7/8 (ELECTRIC & ELECTROMAGNET) NO ASPECT/CHARACTERISTIC/MODIFICATION REASON/EXPLANATION 1 The electric appliances are connected in Allow each electric appliances to be switched on and parallel off independently/Higher voltage One appliaces damage the another can still function 2 Fit fuse at the live wire in the fuse box/Use To stop the flow of current by melting when a high miniature circuit breakers (mcb’s) voltage of electric current flows through the circuit // switches itself off very quickly if the current exceeds 3 Earth connection to the metal case of Earth wire connected to earth, so that when a fault electrical appliances occurs and a current flows through the live wire and the earth wire, the fuse in the live wire will blow and cut off the supply. 4 Use low power lamps / install fluorescent To reduce the energy use lamp Do not waste the electrical energy 5 Regularly cleaning and removing dust from To make sure the appliaces function effectively the air filters of air conditioners 6 Low power lamp Save cost//electric bill 7 High efficiency The room looks brighter//high output power//less power wastage 8 Long life span No need to replace often 9 Low price/cost Save money/cost 10 Smaller surface area The resistance is higher 11 High melting point Not easy to melt 12 Long (coiled) metal To increase the resistance 13 Low rate of oxidation Does not oxidize easily / can be used for a longer period 14 Low resistance Current will increase / more heat will be produced 15 Low resistivity To reduce heat loss in the cables 16 Low rate of thermal expansion The cables will not expand under hot weather 17 Use thin diaphragm Easy to vibrate 18 Use strong material Not easy to break 19 More number of turns of coil Increase the rate of change of magnetic flux linkage // The magnitude of the induced current or is also increased 20 Thicker diameter of wire of coil Reduce the resistance of the coil 21 Using more powerful magnet to increase Increase the rate of change of magnetic flux linkage the strength of the magnetic field //The magnitude of the induced current or induced electromotive force is also increased 22 Change slip rings with commutator To reverse contact with brushes so that the current flow in same direction in external circuit 23 Use stronger magnet To increase the magnetic field strength 24 Use more number of turn for the coil/ Increase the rate of change of magnetic field/increase Increase the speed of rotation the induced current 25 Diameter should be large To reduce the resistance of the cables 26 The rate of expansion should be low So there is less expansion and less sagging in the cables during hot days 27 Use capacitor To smoothen the current produced/to store electric charge 28 Using concave surface soft iron Produce radial magnetic field to ensure smooten rotatation 29 Using a laminated iron core Reduce Eddy current in iron core 30 Thick copper wire Reduce the resistance of the coil 31 Using soft iron for the core Reduce the hysterisis loss. Easy to magnetize and demagnitize 32 Winding the secondary and primary coils Reduce Leakage of Magnetic Flux on top each other. Chapter 9 (RADIOACTIVE) NO ASPECT/CHARACTERISTIC/MODIFICATION REASON/EXPLANATION 1 The half-life should be a few days long This allows for the location to be detected and the radioactive contamination is reduced 2 The source should emit γ particles This enables the radiation to be detected above the ground/high penetrating power 3 The detector should be able to detect γ High ionising particles like α and β particles are particles (low ionising particles) absorbed by the ground 4 Has a long half-life Can be used for a long time hence save cost 5 Emits beta Can penetrate box and liquid and is less dangerous than gamma 6 Solid form Easy to handle and contain. 7 Low ionising power Does not change the state and taste of juice 8 Higher ionising power Easy for the medium to conduct electricity UNDERSTANDING Questions 4. Can you explain why a maximum speed of Form 4 supertanker might need to stop its engine over a distance of about 3 km before it can come to CHAPTER 1 stop? 1. Explain the differences between accuracy and A supertanker at a state of motions and have a consistency of a measuring instrument by maximum velocity. using suitable examples. A supertanker has a larger mass. Accuracy is the ability of the instrument to give A larger mass have a larger inertia. readings close to the actual value. When the engine stop, the supertanker will The value determined is accurate if it is near to continue it state of motions. the actual value So it take a longer distance/time to stop due to The consistency of a measuring instrument is its has a larger inertia. the ability of instrument to record consistent readings for each measurement with little 5. Explain why a softball player moves his hand deviation among readings. backwards while catching a fast moving ball. Other situation: bend our knee after jump? The measurement is consistent if the values determined are close to each other. A soft ball has a high velocity. A soft ball has a high momentum. CHAPTER 2 The soft ball player moves his hand backward 2. To accelerate 2 objects with the same to increase time impact. acceleration, the heavier object needs a bigger The higher the time impact will reduce force. Explain the statement. impulsive force. Higher mass, higher inertia So we will not feel hurt. To accelerate an object, need to overcome the 6. Diagram (a) shows two identical spherical inertia first. plasticine balls before being released from the Therefore, more force is needed for heavier same height. Diagram (b) shows the state of object. the plasticine balls when they hit the wood and the sponge. It was observed that the 3. Can you explain why the passenger thrown plasticine stopped more quickly when it hit the forward when the bus suddenly stop and the wood. head of the passenger were thrown back when Explain the changes in energy that occur from the car started moving? the moment the plasticine ball is released until reaches the position in Diagram (b) When the bus was moving, the passenger were also moving at the same speed as the bus. Before released, the plasticine has Gravitational Potential energy. When the bus stopped, the passengers continued moving. Hence, they were thrown When falling, Gravitational Potential energy foward. changes to Kinetic energy The people in the car tried to remain in their When the plasticine hits the surface of wood, state of rest when the car started moving. the Kinetic energy changes to Heat energy / / Hence, they were thrown back. Sound energy In both situations, the passengers were The energy / work done is use to changes the resisting a change in their state of motion and shape of sponge also known as Inertia. The concept of inertia also known as Newton’s First Law of Motion, which states that “an object will remain at rest or continue with a constant speed in a straight line unless an external forces acting on it” 7. Explain how the forces between the molecules 11. Explain why the boat moves away from the caused the elasticity when the spring is jetty as a boy jumps out of the boat onto the compressed and stretched. river bank. There are two types of force; attraction and When the boy jumps onto the river bank, his repulsive force between the particles of the solid. momentum is forward. When the solid is stretched, the molecules Using the Principle of conservation of displaced away from each other momentum Attractive forces are acting to oppose the the total momentum before and after stretching jumping is equal When the solid is compressed, the molecules The boat moves backward to balance the displaced closer to each other forward momentum Repulsive forces are acting to oppose the 12. Explain why the need of steel structure and compression the separate compartments to build in lorry carrying heavy load. 8. Explain how you can determine the density of cork stopper. The inertia of lorry and load is very big when it is moving Measure the mass of the cork stopper Tie the stopper with string and put it into a The separate compartments make the load divided into smaller mass, thus reducing the measuring cylinder filled with water inertia of each unit. Change in volume of water displaced equal to the The momentum of lorry and load is very big volume of the stopper. when it is moving and produce a bigger Density of stopper = Mass/Volume impulsive force. 9. Using the principle of conservation of The steel structure will prevent the loads from momentum, explain the working principle of smashing into the driver’s compartment the rocket. during emergency braking. Fuel burns in the combustion chamber 13. Why we feel easier to pull the wheel barrow Hot gases expelled at high speed backwards compared to push the load? A large backwards momentum is produced Based on the principle of conservation of The object on the wheel barrow has a weight When we push the wheel barrow there is momentum, the rocket gains forwards momentum of equal magnitude force acting on the ground in the same direction as the weight. 10. Why the boy with mass 40kg slides down the So the total force acting on the ground is the flume when the angle of inclination is 30 o and weight and the force produced when we push remains stationary when the angle of the wheel barrow. inclination is 17.5o.(the frictional force is When we pull the wheel barrow the force 120N) produced is in opposite direction with the weight. Boy slide down when component of weight So the total force acting on the floor is a parallel to the slope is higher than frictional weight less the force produced when we pull force the wheel barrow Resultant force acting to produced acceleration Boy remain stationary when component of weight parallel to the slope is equal to frictional force Resultant force is equal to zero make the boy in force equilibrium CHAPTER 3 balloon 14. Explain why the wooden block move upwards and then float on the water surface when it release from the above of the water surface. Buoyant force increase when the volume of water displace increase. Buoyant force higher than weight of block. Boyant force pushed the wooden block upward. The wooden block then float because the buoyant force is equal to the weight of the wooden block Archimedes principle 15. Exlplain how the brake system operates when the car needs to slow down. When the brake is pressed, a force is applied to the piston and pressure is exerted. Pressure is transmitted uniformly throughout the brake fluid. Force is exerted on the piston of the brake pads Brake pads will press against the brake discs. 16. The toothpaste flows out of it’s tube while squeezing at the bottom end Explain how the toothpaste flows out and name a physics principle related to it. Force is applied to the toothpaste (tube) Will produced a pressure The toothpaste carry the pressure and apply the pressure of the equal magnitude to the whole tube Pascal’s principle 17. Explain how a submarine is able to submerge into deep sea water Valve release air from ballast tank. Sea water flooded ballast tank The weight of water displaced is smaller. Buoyant force < Weight of the submarine 18. Explain why a balloon filled with helium gas rises up in the air. The balloon acted by two forces: Buoyant force and the weight of the balloon The density of helium gas is less than the density of surrounding air Buoyant force equals to the weight of the air displaced by the balloon Buoyant force is higher than the weight of the 19. Diagram shows a copper block and a bowl shape copper sheet of same mass. Explain why the copper block sink in water but the bowl shape copper sheet floats on water two forces act on the copper block and bowl are buoyant force and weight Buoyant force small because small volume // vise versa The average density of cooper sheet is smaller than density of water. Cooper sheet will float. Block sink because weight > Buoyant force Sheet float because weight = Buoyant force 20. Diagram shows a cross-sectional of a wing of a moving aeroplane. The wing of the aeroplane experiences a lift force. Explain why the lift force acts on the wing of the aeroplane. Higher velocity on the upper surface and lower velocity on the lower surface Thus produced lower pressure on the upper surface and higher pressure on the lower surface Lift force = difference in pressure x area of surface Bernoulli’s principle 21. Explain the principle of Insect Piston Spray When the piston is pushed, air is forced out through the jet of gas at a high speed. According to Bernoulli’s Principle, the pressure of the moving air decreases as the speed of the air increases. The higher atmospheric pressure in the insect poison container will push the insect poison liquid up through the narrow metallic tube. 22. Explain how the vacuum cleaner is able to the water absorbed by the fin blade of the remove dust from the floor the fan blow air out of the vent produce a partial vacuum area in the vacuum cleaner difference in pressure occurs/atmospheric pressure is higher than the pressure inside the vacuum cleander forced is exerted in/pushed in the dirt. Bernoulli’s principle 23. Bunsen burner burning with yellow flame . Explain how a blue flame can be produced. High velocity of gas flow in narrow passage of burner creating region of low pressure Higher atmospheric pressure pushes outer air inside and mix with the gas Complete mixture of combustion will produce blue flame Enlarge the orifice to allow more air Bernoulli’s principle 24. The roof of a house being lifted by strong winds. Explain why. The strong wind above the roof is moving very fast While the air in the house is at rest According to Bernoulli’s principle, the higherthe velocity, the lower thepressure pressure inside the house is higher than the outside. a force is generated by the difference in pressure which is strong enough to lift the roof. 25. Explain why the hovercraft moves with constant velocity in terms of the force acting on it The forward force = friction // forward thrust = drag The resultant force is zero The hovercraft is in force in equilibrium CHAPTER 4 26. Water is used as a cooling agent in a radiator. Explain how water is used as a cooling agent in the radiator. Water has high specific heat capacity When water in tube passes through the engine it can absorb large amount of heat energy Once water reach the radiator, the heat of radiator Melting of ice cube will absorb heat(Latent Thesametimethefanintheradiator push heat of fusion) the heat out of the car. The finger has small amount of heat when it is wet and it will be absorbed by the ice cube. 27. Explain how the evaporation process resulting in reduced fluid temperature. • In the water, molecules are constantly moving at different velocities • At water surface, high moving molecules gain high kinetic energy • The bond between molecules overcome and water is released to the air. • Losing of high moving water molecules, water pressure will decrease and thus the temperature decreases 28. According to the principle of thermal equilibrium and the working principle of a thermometer, explain how a doctor can check his patient temperature during medical treatment. Thermometer is placed in the mouth of patient, Heat is transferred from patient’s body to the thermometer. Thermal equilibrium between the thermometer and patient’s body is reached when the net rate of heat transfer is zero. The thermometer and the patient’s body are at the same temperature. The thermometer reading shows the temperature of the patient’s body. 29. Explain the changes which occur in the liquid naphthalene when it is cooled until it changes from the liquid to the solid state. As liquid naphthalene cools, it loses energy to surroundings Its temperature begins to fall until it reaches freezing point 800C At its freezing point, naphthalene begins to solidify as molecules become closely packed. Heat energy is lost to surrounding.(Latent heatof fusion) Temperature remains constant Kinetic energy remains constant 30. Why the ice cube stick to the wet finger. Not to dry Finger? The heat release from water causes it to be 34. The coldest weather experienced in late frozen. winter, when snow began to melt. Explain why? Heat needed to melt snow So the ice cube and finger will stick together Latent heat of fusion is absorbed to convert due to the frozen of water solid into liquid phase Furthermore, finger have a rough surface and More heat is absorbed from the environment it helps the ice stick to our finger 34. Using kinetic theory of gasses, explain how 31. When a few drops of ether hand contact with the pressure increase when the temperature a student, his hands felt cold. Explain how this increase in the pressure cooker. happens. Boiling point of ether is low Molecules moving freely in random motion Temperature of hands higher than the When temperature increase, kinetic temperature of ether energy//velocity increase Cause heat flowing from hand to ether Molecules strike the walls of pressure cooker Ether evaporates // bring the latent heat of more frequently vaporization The rate of change of momentum increase causes the low temperature and cold hands Force exerted on the walls increase, pressure 31. Your body sweats when you are feeling (P = F/A) increase hot.How does sweating helps to cool down your body? 35. In the morning feel hot at the sea .Explain why this phenomenon happens? When we do the activity involving body movement, sweat will be produced. During the day,the land and the sea receive the same amount of heat from the sun Water evaporates from the skin during sweating. Water has a higher specific capacity than the land During evaporation, change of phase of matter from liquid to steam occur. The land is heated to a higher temperature than the sea The heat is needed to change this phase is call the latent heat of vapourisation. The density of the air above the sea is higher than the density of the air above the land So we feel cool when evaporation occur due to the release of heat from our body. The air above the land flows up and the air above the sea flows towards the land Factor influence the process: air velocity, temperature and humidity. CHAPTER 5 36. Tousrist at a beach observing the sunset. 32. We cannot use a cooling system of a refrigerator to cool the hot room. Explain Explain why the tourist can still able to see the why? sun even though it has already set. Cooling system of a refrigerator is smaller Refraction of light ray occurs Less cool air from refrigerator flow out Light travels from less dense to a denser compare to the hot air flow in medium Position of refrigerator is on the floor The light will bend towards the normal The cool air does not flow upward In the observer eyes, the sun is still not setting 33. Why we put the fishes in the ice cube rather as they can still see the image from refraction than cold water? 37. While driving a car on a hot day, you may see Ice melts need heat known as latent heat of a mirage on the road. Explain how mirage fusion occurred. Heat is absorbed from the fish. The layers of air nearer the road warmer. Fish will release heat until 0oC The density of air decrease nearer to the Cold water not experience a change of phase Only process of thermal equilibrium will road surface. The light travel from denser to less dense happen when they in thermal contact. The lower temperature is not 0oC area. The light refract away from the normal When the angle of incidence exceed the critical angle, total internal reflection occurs 38. Diagram shows a ray of light directed 41. It is known that the sky is red during sunset perpendicularly at a side of the semi circular and the formation of rainbow on the sky glass block. The ray passes through the glass always appeared after raining. Explain these block to a point O before leaving the glass phenomena. block. The angle of incidence in the glass block is 30°. Light consisting of seven colours. Red has the longest wave length and the last Explain how total internal reflection occurs in diagram above? to be refracted during sunset. A droplet of water trap in the atmosphere Increase the angle of incidence, then angle of refraction will also increase after raining acts a lens. Light travel through this water droplet and Keep on increasing the angle of incidence until angle of refraction is 90° undergo the process of refraction ,total internal reflection and dispersion of light The angle of incidence is called critical angle occurred. Increase the angle of incidence more than the 42. The sound wave from the train(etc) can be critical angle, the ray will be reflected heard loudly and clearly at night. Why? internally. Air near the ground colder tha above air 39. Explain why a piece of paper burns when Air layer the ground more denser placed under a convex lens aimed towards Sound travel slower in cold air/wavelength hot sun rays. decrease in cold air The parallel rays of the sun will pass through Sound bend toward the observer/sound bend the a convex lens away to normal After entering the lens, the light rays is focused at the principal focus of the lens At the principal focus, the light ray is focused on one small area Heat energy causes an increase in temperature, the paper starts to burn 40. Explain how you would estimate the focal length of a convex lens in your school laboratory. The convex lens is aimed/focused to a distant object (infinity) The screen is adjusted until a sharp image is formed on the screen The distance between the screen and the lens is measured Focal length = distance between the screen and the lens Form 5 47. Descibe the movement of two similiar ships that are located at A and B. Explain? CHAPTER 6 The ship at A will move up and down 43. Explain how the depth of the sea can be Because constructive inteference(Antinodal measured. line) happens at point A • ocean depths can be measured using The ship will remain calm at location B equipment and hydrophone OSK Because destructive inteference(Nodal line) • hydrophone can detect ultrasonic waves in happens at point B water that are emitted CHAPTER 7 • OSK can measure the time after the transmitted and reflected waves back to the 48. Explain the advantages of parallel circuit in a receiver. house wiring system. • The depth can be calculated using the formula A parallel circuit can run several devices using d=(vt)/2 the full voltage of thesupply. 44. Can you explain why the wave front of sea If one device fails, the others willcontinue water will follow the shape of the shore running normally when it approaches the shore? If thedeviceshorts, theotherdeviceswillreceive The depth of the water decreasing as it travel no voltage, preventing overloaddamage. towards the shore. Afailureofonecomponentdoesnotleadtothe The velocity and wavelength is decreasing failureoftheother components. due to the wave travel from the deeper to the shallow area. More componentsmay be added in parallel without the need for morevoltage. The wave will bend and change their direction(refraction occurs). Each electrical appliance in the circuit has it own switch So, wave front of sea water will follow the shape of the shore. 49. Explain why a three pin plug is more suitable compared with a two pin plug. 45. Explain why strong double-glazed glass is used as walls of the observation tower in an Two pin plug has no earth wire while three airport. pin plug has earth wire All particles in a material/matter/glass Using 2 pin plug, if there is leakage of current it vibrate at its natural frequency will also flow through the metal body while using 3 pin plug if there is leakage of current The airplane engine produces noise which it will flow to the ground cause the air to vibrate The person who touches the metal body will Due to resonance, the glass vibrate at a experiences electric shock while using 3 pin higher/maximum amplitude plug, the current will be earthed Need strong glasses to withstand the effect Using 2 pin is not safe to the consumer while of resonance which vibrate with high using3 pinplug is more safer to the consumer amplitude so that it does not brek easily 46. How can when the oprah singer sing can make the glass break. The singer sing with a certain frequency and produce sound energy The energy is transferred to the glass Resonance occures when the sound frequency made by the singer is the same as the natural frequency of the glass The glass will vibrate with maximum amplitude Increase in energy transferred may cause the glass to break 50. What happen to the candle flame when it CHAPTER 8 place between 2 metal plate supply with Extra High Tension (EHT). 54. The acceleration of a magnet that drops vertically into a solenoid is much smaller than Candle flame spread into two the gravitational acceleration. Explain the Heat from candle split neutral air molecules statement. into + and - ions Magnetic flux change in the solenoid Positive charge will attracted to negative plate Induced current generates in the solenoid Direction of induced current always flows while negative charge will atracted to positive plate in the direction to generate magnetic pole Candle flame spread wider to negative plate to oppose the pole of the falling magnet. because positive charge is more haviear than (Lenz’s law) negative charge Therefore, acceleration is lower 51. Toaster T marked 240 V, 650 W and toaster U 55. Explain how the electromagnet crane canbe marked 240 V, 840 W. used to lift scrapmetal. Determine Which toaster has a heating element with a smaller resistance. Current flow through the solenoid, magnetic field is produced • toaster U • both the toaster has equal voltage Soft iron core will be magnetized • Power = V2/R The scrap metal attracted to the iron core • toaster with a higher power has a heating No current flow, soft iron demagnetized element with a smaller resistance and metal scrap fall down 52. Explain why the bulb connected to two dry 56. Most of our electric energy comes from cells lights up brighter than one bulb hydroelectric power stations and thermal connected to one dry cell. power station. These power stations are connected by cables to transmit electricity to The two dry cells are connected in parallel users in industries, offices, schools and The effective e.m.f. remains the same houses. This system is called the national grid The effective internal resistance of the two network. Explain briefly the importance of the national cells is smaller grid network system in distributing electric A larger current will flow through the bulb to energy to the users. make it brighter The electrical supply is continuous, although there is faulty in one of the 53. A battery consists of two 1.5 V dry cells power station connected to a bulb labeled 2.5V, 0.3 A. Found that the bulb is lit with a normal The electrical energy from other station is brightness when the switch is turned on. directed to the affected areas Explain why the bulb is lit with a normal brightness even EMF of battery is greater than The electrical energy from other area is that metol. directed to the areas that need more energy • battery has a internal resistance • some of the battery EMF is lost to overcome The overall cost of production of electricity can be reduced internal resistance • So, voltage supplied to the bulb is almost similar to voltage needed to light the bulb 57. Diagram shows the pattern of magnetic field 59. Explain how the generator works to produce formed when current flows in a coil. direct current. Explain why the magnetic field strength is greater at the center compared to the edge. rotate the coil in clockwise direction the coil cut across the magnetic field The direction of the magnetic field on the left current is induced in the coil coil is anti clockwise The commutator change the direction in the The direction of the magnetic field on the coil so that the direction of current in external right coil is clockwise circuit always the same. As the result the magnetic fields in the 60. Explain how the speed of coil (force) of middle of the coil are in the same direction, generator can be increased. ie upward. Increased input current So the magnetic field will be stronger in the Increase strength of magnetic field middle. Increase length of conductor 58. Using the concept of the magnetic effect of 61. Explain how to increase induced current in a an electric current, explain with the aid of generator. diagrams how forces are produced on a wire in the coil of direct current electric motor? Increase the speed of conductor Increase strength of magnetic field The magnets produce a magnetic field / Increase length of conductor diagram 62. Explain the working principle of a transformer. The current in the wire produces a magnetic field / diagram When a.c. voltage is supplied to primary coil, (alternating current will flow) and The two magnetic fields interact/combine to form a resultant / catapult field / diagram The soft iron core is magnetized. The magnet produced varies in magnitude The motor will rotate due to the differences of force produce//turning effect from this and direction. two forces This causes a changing magnetic flux pass through the secondary coil. An induced EMF across the secondary coil is SN produced 63. Explain the working principle of an electric bell. When the bell is pressed, a current flows in the coils of the electromagnet, causing the electromagnet to be magnetized. The magnetized electromagnet attracts the soft-iron armature, causing the hammer to strike the gong. The movement of the armature breaks the circuit and causes the electromagnet to lose it magnetism. The light spring pulls the armature back, remaking the contact and completing the circuit again. The cycle is repeated so long as the bell push is pressed and continuous ringing occurs. 64. Explain how to increase the sensitivity of a CHAPTER 10 moving coil meter? 68. Radioisotope Strontium-90 is used to Increase the strength of magnetic field measure the thickness of paper in a paper Increase the number of turns of coil industry Explain how Strontium-90 is used Increase the stiffness of hairsprings to measure the thickness piece of paper? Decrease the mass of the coil and pointer Put the radioactive source opposite the CHAPTER 9 detector 65. Explain how the green shadow produced in Detector is connected to the thickness the CRO screen? indicator 6V heater supply produced electron on it Detector detect the reading of the changes in surface counts When 3 kV power supply are connected Thickness is measured with the thickness Cathode rays/electron accelerate in a straight indicator line. If the reading of the detector is less than the Cathode rays carry kinetic energy and specified value, the thickness of the paper Converts to light energy when they hit the is too tick/ vice versa screen. 69. Nuclear fission produces a chain reaction. 66. Explain why the bulb light up at night Describe how the chain reaction occurs in a nuclear fission of an atom of Uranium- 235. At night resistance LDR increases VBE increases (higher than 0.7 V for Si) Neutron bombarded a uranium nucleus and Ib increases and switch on transistor produced three neutral neutron Ic, increases and lights up bulb The new neutron bombarded a new uranium 67. Explain how to increase rate of thermionic nucleus emission. For every reaction, the neutrons produced Increase the temperature of metal will generate a chain reaction Increase surface area Copper has higher rate of thermionic emission Diagram of chain reaction then iron. 70. Alpha particles can observed by using clod Coat with matal oxides. Matal oxides emit chamber. Explain why the tracks formed is thick and straight tracks. electron at lower temperature. The tracks are thick due to strong ionising effect of alpha particles. A lot of alcohol droplets are formed on the ions produced along the track. The tracks are straight because the alpha particles are not easily deflected due to its greater mass 71. Explainhow radioisotopes can be used to detect the location of the leakage Radioisotope is injectedinto the pipe The water in the pipe flow with the radioisotope G-M tube as detector is used to find the leakage across the pipe Reading on detector increases when near a leakage Chap. ITEM KNOWLEDGE/DEFINATION 1 Derived quantity DEFINATION 1 Base quantity A physical quantity derived from combinations of base quantities through 1 Scalar quantity multiplication or division or both multiplication and division. 1 Vector quantity A physical quantity that cannot be defined in terms of other quantities. 1 Physical quantity 1 Error A physical quantity that has magnitude only. 1 Random error A physical quantity that has magnitude and direction. 1 Systematic error 1 Zero error A quantity that can be measured. 1 Sensitivity The difference between the measured value and the actual value. 1 Accuracy 1 Consistency Error due to mistakes made when making measurement either through incorrect positioning of the eye or the instrument when making measurement. Repeat and 1 Hypothesis calculate average reading to minimise. An error which may be due to the error in the calibration of an instrument. 1 Inference 1 Variable Error due to non-zero reading when the actual reading should be zero 1 Prefix The ability of a measuring instrument to detect a small change in the quantity to be measured. 1 Scientific How close the measurement made is to the actual value. notation/Standard 2 form The ability of the instrument to measure a quantity with little or no deviation 2 Distance among measurements. 2 Displacement A statement of an expected outcome that usually states the relationship between Speed two or more variables intended to be given a direct experimental test. 2 An initial interpretation or explanation concerning the observation. 2 Velocity 2 Acceleration A physical quantity that can be varied in an experiment. There are three types of Formula variables; manipulated variable, responding variable and fixed variable. 2 A word, letter or value used to simplify the description of the magnitude of a Inertia physical quantity that either very big or very small. 2 A way to write a numerical magnitude in the form A x 10", where 1 A < 10 and n is Newton’s First Law an integer. 2 of Motion Newton’s Second The total length of the path travelled from one location to another. Law of Motion The length of the straight line connecting the two locations, in a specified direction. The distance travelled per unit time. It is also defined as the rate of change of distance. The speed in specified direction. The rate of change of displacement. The rate of change of velocity. v = u + at v2 = u2+ 2as s = ut + at2 s = (u+v)t a= The inertia of an object is the tendency of the object to remain at rest or, if moving, to continue its uniform motion in a straight line. An object will remain at rest or continue with a constant speed in a straight line unless an external forces acting on it The acceleration of a body is parallel and directly proportional to the net force and inversely proportional to the mass m, i.e., F = ma. Physics Tips SPM 2020 AG#1 2 Newton’s Third Law The mutual forces of action and reaction between two bodies are equal, opposite of Motion and collinear. 2 Momentum The momentum of an object is defined as the product of its mass and its velocity. [ Momentum=mv ]/ms-1 2 Principle of The total momentum if a system remains constant in the absence of external Conservation of force. Momentum 2 Elastic collision Momentum, kinetic energy and total energy are conserved. [ m1u1+m2u2=0 ] 2 Inelastic collision Momentum and total energy are conserved but the kinetic energy after the collision is less than the kinetic energy before the collision. [ m1u1+m2u2= (m1+m2)v ] 2 Force Constant F: Acceleration inversely proportional to mass. Constant m: Acceleration directly proportional to force. [ F=ma ]/N 2 Impulse The quantity of impulsive force multiplied by time. [ Impulse=Ft/Ns ]//[ Impulse=mu-mv]/cms-1 2 Impulsive force The rate of change of momentum. [ (mv-mu)/t ] 2 Resultant force A single force that represents the combined effect of two or more forces by taking into account both the magnitude and the direction of the forces. 2 Mass The amount of matter in an object. 2 Weight The force of gravity acting on an object. [ Weight= mg ]/N 2 Work The product of an applied force and displacement of an object in the direction of 2 Power the applied force. [ Work=Fs ]/J Rate of work done. [ P=Work done/t ]/W//Js-1 2 Energy The capacity of a system to enable it to do work. 2 Kinetic energy The energy of an object due to its motion. [ KE= mv2] /J 2 Gravitational The energy of an object due to its higher position in the gravitational field. potential energy [ GPE= mgh ]/J 2 Gravitational The acceleration of an object due to the pull of the gravitational force. acceleration 2 Gravitational field A region in which an object experiences a force due to the gravitational attraction towards the centre of the Earth. 2 Gravitational field The gravitational force acting on a mass of 1 kg placed at a point in the gravitational strength field. 2 Free fall The motion when an object is acted upon by a gravitational force in the gravitational field. 2 Elastic potential The energy stored in an object when it is extended or compressed by a force. energy [ EPE= Fx ]/J 2 Principle of Energy can be transformed to one form to another but i cannot be destroyed or Conservation of created. energy The percentage of the input energy that is transformed into useful energy. 2 Efficiency Efficiency = x 100% 2 Non-renewable An energy resource that cannot be replaced once it has been used. energy resource Physics Tips SPM 2020 AG#1 2 Renewable energy An energy resource that is continually replaced and will not run out. resource 2 Elasticity A property of matter that enables an object to return to its original size and shape 2 Hooke's law when the force that was acting on it is removed. 3 Pressure The extension of a spring is directly proportional to the applied force provided the elastic limit is not exceeded. Force acting per unit area on the surface. [ Pressure = ]/Pa//Nm-2 3 Pressure in liquid [ Pressure = phg ]/ Pa//Nm-2 Pascal's principle Pressure applied to an enclosed liquid is transmitted uniformly to every part of the 3 liquid (and to the walls of the container of the liquid) 3 Archimedes' For a body wholly or partially immersed in a fluid, the upward buoyant force acting principle on the body is equal to the weight of the fluid it displaces. 3 Bernoulli's principle Where the speed of a fluid is high, the pressure is low and vice versa. 3 Buoyant force An upward force, resulting from an object being wholly or partially immersed in a fluid. [ BF = Vpg ]/N 3 Atmospheric The pressure exerted by the atmosphere on the surface of the Earth as well as all pressure objects on the Earth. 4 Temperature The measure of the degree of hotness of an object. 4 Thermometer An instrument that measures temperature or the degree of hotness. 4 Boiling point The temperature at which a substance changes from a liquid to a gaseous state, 4 Melting point where the change occurs throughout the liquid. The temperature at which a substance changes its state from a solid to a liquid. 4 Thermal equilibrium A condition where two objects in thermal contact have no net flow of heat energy between each other. 4 Heat capacity The amount of heat that must be supplied to a body to increase its temperature by 1°C. [ J°C-1 ] 4 Specific heat The amount of heat that must be supplied to increase the temperature by 1°C for a capacity mass of 1 kg of the substance. [c = J°C-1 kg-1 ] 4 Latent heat The heat absorbed or the heat released at constant temperature during a change of phase. 4 Specific latent heat The amount of heat required to change 1 kg of a substance from the solid to liquid of fusion phase without a change in temperature. [ Q=mLf ] /J 4 Specific latent heat The amount of heat required to change 1 kg of a substance from the liquid to of vaporisation gaseous phase without a change in temperature. [ Q=mLv ] /J 4 Boyle's law For a fixed mass of gas, the pressure of the gas is inversely proportional to its volume when the temperature is kept constant. [PV=Constant ] 4 Charles' law For a fixed mass of gas, the volume of the gas is directly proportional to its absolute temperature when its pressure is kept constant. [ = Constant ] 4 Pressure law For a fixed mass of gas, the pressure of the gas is directly proportional to its absolute temperature when its volume is kept constant. [ = Constant ] 5 Concave lens A lens that is thinnest at its centre. It causes parallel rays of light to diverge after 5 Convex lens passing through this lens. A lens that is thickest at its centre. It causes parallel rays of light to converge after passing through this lens. Physics Tips SPM 2020 AG#1 5 Angle of incidence The angle between the incident ray and the normal. 5 Angle of reflection The angle between the reflected ray and the normal. 5 Focal length, f The distance between the centre of a lens to its focal point. 5 Focal point, F A point to which all rays parallel to the principle axis converge or appear to diverge from, after reflection by the mirror(refraction by lens). 5 Refraction of light The bending of a light ray at the boundary as it travels from one medium to another. 5 Critical angle The angle of incidence in the denser medium when the angle of refraction in the less dense medium is equal to 90°. 5 Total internal The condition where the angle of incidence, i is increased further so that it is reflection greater than the critical angle, c. The light is no longer refracted but is internally reflected. 5 Real depth The distance of the real object, 0 from the surface of the water or medium. 5 Apparent depth The distance of the virtual image, I from the surface of the water. 5 Real image An image that can be displayed on a screen. 5 Virtual image An image that can be seen by the observer but not be displayed on a screen. 6 Period, T The time taken to complete one oscillation. 6 Frequency, f The number of complete oscillations in one second. [ f = 1/t ]/s-1 6 Amplitude, a The maximum displacement from the mean position. 6 Transverse wave A wave in which the particles of the medium oscillate in the direction perpendicular to the direction in which the wave moves. 6 Longitudinal wave A wave in which the particles of the medium oscillate in the direction parallel to the direction in which the wave moves. 6 Wave A way of transmission of energy from one point to another without transfering of matter. 6 In Phase Waves that vibrate in same direction and same distance from the equilibrium position. 6 Wavefront A line of plane which the oscillation of every points on it are in phase and the points are at same distance from the source of the waves. 6 Wavelength The horizontal distance between two adjacent points of the same phase on a wave. [ v = fλ ]/ms-1 6 Damping When the system loses energy to the surrounding in the form of heat. Amplitude decreases. 6 Resonance When a system is made to oscillate at a frequency equivalent to its natural frequency by an external force. 6 Reflection of waves Angle of reflection = Angle of incident Same speed, wavelength, frequency but direction of propagation of wave changes. Amplitude decreases due to heat lost. 6 Refraction of waves Refraction of waves occurs when there is change of direction of the propagation of waves travelling from a medium to another medium due to a change of speed. From shallow region(denser) to deep region (less dense) Speed increases Wavelength increases Wave refracted from normal Frequency constant Physics Tips SPM 2020 AG#1 6 Diffraction of waves Spreading of waves after passing through a gap or a small obstacle. 6 Interference of Caused by superposition of waves from 2 coherent sources, resulting in waves constructive and destructive interference. [ λ= ] 6 Principle of When 2 waves interfer, the resultant displacement of the waves is the sum of the Superposition displacements of the individual waves. A point where a contructive interference occurs. 6 Antinode 6 Node A point where a destructive interference occurs. 6 Coherent waves Waves having the same frequency, wave length,amplitude and in phase. 6 Electromagnetic A group of waves with similar natures. The members of the electromagnetic spectrum spectrum arranged in increasing frequencies (decreasing wavelengths) are radio waves, microwaves, infrared rays, visible light, ultraviolet rays, X-rays and gamma rays. 6 Angle of refraction The angle between the refracted ray and the normal. 7 Electric current The rate of charge flow in a circuit. [ I = ]/A 7 Potential difference Energy needed to move 1 electric charge,C around a complete circuit. (Between 2 points) [ V = ]/V 7 Resistance Ratio of potential difference to current. [ R = ]/ Ω 7 Power The rate of work done. 7 Electric field A region in which an electric charge experiences an electrostatic force. 7 Ohm’s Law The current in a conductor is proportional to the potential differences across the ends of the conductor if the psysical conditions remain constant. Psysical conditions: I Length Temperature Cross-sectional area Types of materials V 7 Ohmic Conductor Conductor which obey Ohm’s Law. 7 Series circuit A circuit where all the electrical components are connected one end after the other to a cell to form a single pathway for a current to flow. 7 Parallel circuit A circuit where all the electrical components are connected side by side and their corresponding ends are joined together to a cell to form separate and parallel paths for a current to flow. 7 Open Circuit The battery is connected to a voltmeter only. (Test EMF) 7 Electrical energy The energy supplied by source of electricity when current flows in a close circuit. [ E = Pt ]/J 7 Electric power The rate of transfer of electrical energy. 7 Electromotive force Energy needed to move 1 electric charge,C around a complete circuit. (Between 2 terminals) 7 Internal resistance The resistance against the moving charge due to the electrolyte in the cell. 8 Magnetic field A region in which the magnetic materials experience a force. 8 Electromagnet A device which its magnetism is produced by electric current. 8 Right-hand grip rule Determine the magnetic pole of a current-carrying solenoid/direction of magnetic field. Physics Tips SPM 2020 AG#1 8 Fleming’s Left hand Determine the direction of motion of current-carrying conductor in a magnetic rule field. 8 Fleming’s Right hand Determine the direction of induced current. rule 8 Direct current motor Convert electric energy into mechanical energy. 8 Electromagnetic Production of electromotive force in a conductor due to a change in magnetic flux induction linking the conductor. 8 Transformer A device which steps up or steps down alternating current voltages. 8 Direct current A current which flows in one direction only. 8 Alternating current A current which flows to and fro in two opposite directions in a circuit. It changes its direction periodically. Used to transfer current as its voltage can be step up or down easily by tansformer. 8 Electrical power lost P = VI = I2R 8 Faraday's law The magnitude of the induced e.m.f, is directly proportional to the rate of change of the magnetic flux. 8 Lenz's law The direction of the induced current always oppose the change producing it. 8 National Grid A network system of cables which connects all the power stations and substations Network in the country to the consumers in a closed network to transmit electricity. 9 Thermionic emission The emission of electrons from the surface of a heated metal. 9 Cathode rays Beam of fast-moving electrons. 9 Semiconductor A material which can conduct electricity better than insulator, but not as well as conductor. 9 Doping A process of adding a small amount of specific impurities called dopants to semiconductors to increase their conductivity. 9 Emitter current The current that flows through the emitter terminal of a transistor. It is equal to the sum of the base current and the collector current. 9 Cathode ray An instrument that converts electronic and electrical signals to a visual display. oscilloscope 9 Maltese Cross tube A special cathode ray tube with a Maltese Cross in it which is used to investigate the properties of cathode rays. 9 Diode A device that allows current to flow in one direction only. 9 Forward biased A state when a diode allows current to flow. 9 Reverse biased A state when a diode does not allow current to flow 9 Rectification A process to convert an alternating current into a direct current by using a diode or diodes. 9 Half-wave A process where only one half of every cycle of an alternating current is made to rectification flow in one direction only. 9 Full-wave A process where both halves of every cycle of an alternating current is made to rectification flow in the same direction using bridge rectifier. 9 Transistor An electronic device which has three terminals labelled as base, collector and emitter. 9 Base current The current that flows through the base terminal of a transistor. Physics Tips SPM 2020 AG#1 9 Collector current The current that flows through the collector terminal of a transistor. It will only flow when a suitable base current flows through the circuit. 9 Capacitor A device used for storing charges and to smooth out output current in a rectifier 9 Logic gate circuit. Switching circuit that is applied in computers and other electronic devices. 10 Nucleus A very small core of an atom which contains most of the mass and all of the positive charge of the atom. 10 Proton number The total number of protons in a nucleus. 10 Nucleon A subatomic particle found in the nucleus. 10 Nucleon number The total number of protons and neutrons in a nucleus. 10 Isotopes Atoms of an element which have the same proton number but different nucleon 10 Radioisotope numbers. Unstable isotopes which decay and give out radioactive emissions. 10 Radioactivity The spontaneous disintegration of an unstable nucleus accompanied by the 10 Alpha particle emission of energetic particles or photons. Helium nucleus emitted by an unstable nucleus. 10 Beta particle High energy electron emitted by an unstable nucleus. 10 Gamma rays Electromagnetic waves with very high frequency and short wavelength. 10 Alpha decay A radioactive decay which emits an alpha particle. [→ + ] 10 Beta decay A radioactive decay which emits a beta particle. [ →+ ] 10 Gamma decay A radioactive decay which emits a gamma ray photon. [ → +] 10 Half-life The time taken for the number of undecayed nuclei to be reduced to half of its 10 Nuclear energy original number.ie. mass, number of nuclei and activity. Energy released by a nuclear reaction as a result of a mass defect. [ E = mc2 ] 10 Nuclear fission The splitting of a heavy nucleus into two or more lighter nuclei. 10 Nuclear fusion The combining of two or more lighter nuclei to form a heavier nucleus. 10 Chain reaction A self-sustaining reaction in which the products of a reaction can initiate another 10 Somatic effect similar reaction. The effect of radiation that appears in a person exposed to radiation. 10 Genetic effect The effect of radiation that appears in the future generations of the exposed person as a result of radiation damage to reproductive cells. Base Quantity S.I. Unit 1. Length m 2. Mass kg 3. Time s 4. Current A 5. Temperature K Physics Tips SPM 2020 AG#1 PAPER 3 Precautions: 1. The position of eye must be pependicular to the scale reading to aviod error due to parallax error ( N/A if instrument is digital stopwatch) 2. Check the voltmeter for zero error and make zero adjustment 3. Make sure elastic strings are stretched at constant length.(Force and acceleration) 4. Stir the water gently with the heater to ensure that heat is distributed uniformly to all part of the water.(Heat) 5. Make sure the insulating jacket is covered all part of the beaker to prevent heat loss.(Heat) 6. Assume no heat loss to the surrounding. (Heat) 7. Make sure the experiment is conducted in a dark room.(Light) 8. The wires and electrical components should be connected tightly to avoid current loss.(Electric) 9. The circuit should be switched off when not taking readings to avoid overheating.(Electric) 10. Use small value of current to avoid the wire from getting too hoteasily.(Electric) Physics Tips SPM 2020 AG#1
- DOI: 10.1016/S0065-2687(08)60079-5
- Corpus ID: 118796259
The Physics of Cloud Modification
- J. E. McDonald
- Published 1958
- Physics, Environmental Science
- Advances in Geophysics
24 Citations
Weather‐climate modification, linking remote sensing and in-situ data of cloud properties, on the regulation of climate: a sulfate particle feedback loop involving deep convection, state water survey division, is there a positive feedback between arctic stratus and arctic sea ice changes, simulation of meteorological fields for icing applications at the summit of mount washington, reflectivity, rain rate, and kinetic energy flux relationships based on raindrop spectra, particle impact and breakup in aircraft measurement, mechanism of cloud droplet motion under sound wave actions, the snows of interior alaska, 80 references, on the physics of clouds and precipitation, a preliminary quantitative analysis of precipitation mechanisms, the problem of artificial control of rainfall on the globe1, the growth of cloud drops in uniformly cooled air, an inherent precipitation mechanism in cumulus clouds, turbulence in clouds as a factor in precipitation, the artificial stimulation of precipitation by means of dry ice, the production of showers by the coalescence of cloud droplets, advances in radar weather, observations on the formation and spread of precipitation in convective clouds, related papers.
Showing 1 through 3 of 0 Related Papers
SPM Physics
SPM Form 4 Form 5 Revision Notes and Videos
Direct Current Motor
- An electric motor converts electrical energy to kinetic energy.
- The diagram above shows the structure of a simple direct current motor (DC motor).
- It consists of a rectangular coil of wire placed between 2 permanent magnets.
- The coil is soldered to a copper split ring known as a commutator. 2 carbon brushes are held against the commutator.
- The function of the brush is to conduct electricity from the external circuit to the coil and allow the commutator to rotate continuously.
- The function of the commutator is to change the direction of the current in the coil and hence change the direction of the couple (the 2 forces in opposite direction) in every half revolution. This is to make sure that the coil can rotate continuously.
- The operation principle of a direct current motor is explained in detail in the second Youtube video below.
Recommended Video SPM Physics – Direct Current Motor
In this video, our tutor explains the important point that SPM students need to know to prepare for their exam.
This video shows how the commutator changes the direction of the current in the coil, and hence change the direction of the force in every half revolution. Pay attention to the change of direction of the force in every half revolution.
This is a very long video, discussing how the force is produced by the interaction between a current-carrying conductor in a magnetic field and its application in the direct current motor.
External Resources
Physics animation, external link.
- Search Menu
Sign in through your institution
- Browse content in Arts and Humanities
- Browse content in Archaeology
- Anglo-Saxon and Medieval Archaeology
- Archaeological Methodology and Techniques
- Archaeology by Region
- Archaeology of Religion
- Archaeology of Trade and Exchange
- Biblical Archaeology
- Contemporary and Public Archaeology
- Environmental Archaeology
- Historical Archaeology
- History and Theory of Archaeology
- Industrial Archaeology
- Landscape Archaeology
- Mortuary Archaeology
- Prehistoric Archaeology
- Underwater Archaeology
- Zooarchaeology
- Browse content in Architecture
- Architectural Structure and Design
- History of Architecture
- Residential and Domestic Buildings
- Theory of Architecture
- Browse content in Art
- Art Subjects and Themes
- History of Art
- Industrial and Commercial Art
- Theory of Art
- Biographical Studies
- Byzantine Studies
- Browse content in Classical Studies
- Classical History
- Classical Philosophy
- Classical Mythology
- Classical Numismatics
- Classical Literature
- Classical Reception
- Classical Art and Architecture
- Classical Oratory and Rhetoric
- Greek and Roman Epigraphy
- Greek and Roman Law
- Greek and Roman Papyrology
- Greek and Roman Archaeology
- Late Antiquity
- Religion in the Ancient World
- Social History
- Digital Humanities
- Browse content in History
- Colonialism and Imperialism
- Diplomatic History
- Environmental History
- Genealogy, Heraldry, Names, and Honours
- Genocide and Ethnic Cleansing
- Historical Geography
- History by Period
- History of Emotions
- History of Agriculture
- History of Education
- History of Gender and Sexuality
- Industrial History
- Intellectual History
- International History
- Labour History
- Legal and Constitutional History
- Local and Family History
- Maritime History
- Military History
- National Liberation and Post-Colonialism
- Oral History
- Political History
- Public History
- Regional and National History
- Revolutions and Rebellions
- Slavery and Abolition of Slavery
- Social and Cultural History
- Theory, Methods, and Historiography
- Urban History
- World History
- Browse content in Language Teaching and Learning
- Language Learning (Specific Skills)
- Language Teaching Theory and Methods
- Browse content in Linguistics
- Applied Linguistics
- Cognitive Linguistics
- Computational Linguistics
- Forensic Linguistics
- Grammar, Syntax and Morphology
- Historical and Diachronic Linguistics
- History of English
- Language Acquisition
- Language Evolution
- Language Reference
- Language Variation
- Language Families
- Lexicography
- Linguistic Anthropology
- Linguistic Theories
- Linguistic Typology
- Phonetics and Phonology
- Psycholinguistics
- Sociolinguistics
- Translation and Interpretation
- Writing Systems
- Browse content in Literature
- Bibliography
- Children's Literature Studies
- Literary Studies (Asian)
- Literary Studies (European)
- Literary Studies (Eco-criticism)
- Literary Studies (Romanticism)
- Literary Studies (American)
- Literary Studies (Modernism)
- Literary Studies - World
- Literary Studies (1500 to 1800)
- Literary Studies (19th Century)
- Literary Studies (20th Century onwards)
- Literary Studies (African American Literature)
- Literary Studies (British and Irish)
- Literary Studies (Early and Medieval)
- Literary Studies (Fiction, Novelists, and Prose Writers)
- Literary Studies (Gender Studies)
- Literary Studies (Graphic Novels)
- Literary Studies (History of the Book)
- Literary Studies (Plays and Playwrights)
- Literary Studies (Poetry and Poets)
- Literary Studies (Postcolonial Literature)
- Literary Studies (Queer Studies)
- Literary Studies (Science Fiction)
- Literary Studies (Travel Literature)
- Literary Studies (War Literature)
- Literary Studies (Women's Writing)
- Literary Theory and Cultural Studies
- Mythology and Folklore
- Shakespeare Studies and Criticism
- Browse content in Media Studies
- Browse content in Music
- Applied Music
- Dance and Music
- Ethics in Music
- Ethnomusicology
- Gender and Sexuality in Music
- Medicine and Music
- Music Cultures
- Music and Religion
- Music and Media
- Music and Culture
- Music Education and Pedagogy
- Music Theory and Analysis
- Musical Scores, Lyrics, and Libretti
- Musical Structures, Styles, and Techniques
- Musicology and Music History
- Performance Practice and Studies
- Race and Ethnicity in Music
- Sound Studies
- Browse content in Performing Arts
- Browse content in Philosophy
- Aesthetics and Philosophy of Art
- Epistemology
- Feminist Philosophy
- History of Western Philosophy
- Meta-Philosophy
- Metaphysics
- Moral Philosophy
- Non-Western Philosophy
- Philosophy of Science
- Philosophy of Language
- Philosophy of Mind
- Philosophy of Perception
- Philosophy of Action
- Philosophy of Law
- Philosophy of Religion
- Philosophy of Mathematics and Logic
- Practical Ethics
- Social and Political Philosophy
- Browse content in Religion
- Biblical Studies
- Christianity
- East Asian Religions
- History of Religion
- Judaism and Jewish Studies
- Qumran Studies
- Religion and Education
- Religion and Health
- Religion and Politics
- Religion and Science
- Religion and Law
- Religion and Art, Literature, and Music
- Religious Studies
- Browse content in Society and Culture
- Cookery, Food, and Drink
- Cultural Studies
- Customs and Traditions
- Ethical Issues and Debates
- Hobbies, Games, Arts and Crafts
- Natural world, Country Life, and Pets
- Popular Beliefs and Controversial Knowledge
- Sports and Outdoor Recreation
- Technology and Society
- Travel and Holiday
- Visual Culture
- Browse content in Law
- Arbitration
- Browse content in Company and Commercial Law
- Commercial Law
- Company Law
- Browse content in Comparative Law
- Systems of Law
- Competition Law
- Browse content in Constitutional and Administrative Law
- Government Powers
- Judicial Review
- Local Government Law
- Military and Defence Law
- Parliamentary and Legislative Practice
- Construction Law
- Contract Law
- Browse content in Criminal Law
- Criminal Procedure
- Criminal Evidence Law
- Sentencing and Punishment
- Employment and Labour Law
- Environment and Energy Law
- Browse content in Financial Law
- Banking Law
- Insolvency Law
- History of Law
- Human Rights and Immigration
- Intellectual Property Law
- Browse content in International Law
- Private International Law and Conflict of Laws
- Public International Law
- IT and Communications Law
- Jurisprudence and Philosophy of Law
- Law and Politics
- Law and Society
- Browse content in Legal System and Practice
- Courts and Procedure
- Legal Skills and Practice
- Legal System - Costs and Funding
- Primary Sources of Law
- Regulation of Legal Profession
- Medical and Healthcare Law
- Browse content in Policing
- Criminal Investigation and Detection
- Police and Security Services
- Police Procedure and Law
- Police Regional Planning
- Browse content in Property Law
- Personal Property Law
- Restitution
- Study and Revision
- Terrorism and National Security Law
- Browse content in Trusts Law
- Wills and Probate or Succession
- Browse content in Medicine and Health
- Browse content in Allied Health Professions
- Arts Therapies
- Clinical Science
- Dietetics and Nutrition
- Occupational Therapy
- Operating Department Practice
- Physiotherapy
- Radiography
- Speech and Language Therapy
- Browse content in Anaesthetics
- General Anaesthesia
- Browse content in Clinical Medicine
- Acute Medicine
- Cardiovascular Medicine
- Clinical Genetics
- Clinical Pharmacology and Therapeutics
- Dermatology
- Endocrinology and Diabetes
- Gastroenterology
- Genito-urinary Medicine
- Geriatric Medicine
- Infectious Diseases
- Medical Toxicology
- Medical Oncology
- Pain Medicine
- Palliative Medicine
- Rehabilitation Medicine
- Respiratory Medicine and Pulmonology
- Rheumatology
- Sleep Medicine
- Sports and Exercise Medicine
- Clinical Neuroscience
- Community Medical Services
- Critical Care
- Emergency Medicine
- Forensic Medicine
- Haematology
- History of Medicine
- Browse content in Medical Dentistry
- Oral and Maxillofacial Surgery
- Paediatric Dentistry
- Restorative Dentistry and Orthodontics
- Surgical Dentistry
- Browse content in Medical Skills
- Clinical Skills
- Communication Skills
- Nursing Skills
- Surgical Skills
- Medical Ethics
- Medical Statistics and Methodology
- Browse content in Neurology
- Clinical Neurophysiology
- Neuropathology
- Nursing Studies
- Browse content in Obstetrics and Gynaecology
- Gynaecology
- Occupational Medicine
- Ophthalmology
- Otolaryngology (ENT)
- Browse content in Paediatrics
- Neonatology
- Browse content in Pathology
- Chemical Pathology
- Clinical Cytogenetics and Molecular Genetics
- Histopathology
- Medical Microbiology and Virology
- Patient Education and Information
- Browse content in Pharmacology
- Psychopharmacology
- Browse content in Popular Health
- Caring for Others
- Complementary and Alternative Medicine
- Self-help and Personal Development
- Browse content in Preclinical Medicine
- Cell Biology
- Molecular Biology and Genetics
- Reproduction, Growth and Development
- Primary Care
- Professional Development in Medicine
- Browse content in Psychiatry
- Addiction Medicine
- Child and Adolescent Psychiatry
- Forensic Psychiatry
- Learning Disabilities
- Old Age Psychiatry
- Psychotherapy
- Browse content in Public Health and Epidemiology
- Epidemiology
- Public Health
- Browse content in Radiology
- Clinical Radiology
- Interventional Radiology
- Nuclear Medicine
- Radiation Oncology
- Reproductive Medicine
- Browse content in Surgery
- Cardiothoracic Surgery
- Gastro-intestinal and Colorectal Surgery
- General Surgery
- Neurosurgery
- Paediatric Surgery
- Peri-operative Care
- Plastic and Reconstructive Surgery
- Surgical Oncology
- Transplant Surgery
- Trauma and Orthopaedic Surgery
- Vascular Surgery
- Browse content in Science and Mathematics
- Browse content in Biological Sciences
- Aquatic Biology
- Biochemistry
- Bioinformatics and Computational Biology
- Developmental Biology
- Ecology and Conservation
- Evolutionary Biology
- Genetics and Genomics
- Microbiology
- Molecular and Cell Biology
- Natural History
- Plant Sciences and Forestry
- Research Methods in Life Sciences
- Structural Biology
- Systems Biology
- Zoology and Animal Sciences
- Browse content in Chemistry
- Analytical Chemistry
- Computational Chemistry
- Crystallography
- Environmental Chemistry
- Industrial Chemistry
- Inorganic Chemistry
- Materials Chemistry
- Medicinal Chemistry
- Mineralogy and Gems
- Organic Chemistry
- Physical Chemistry
- Polymer Chemistry
- Study and Communication Skills in Chemistry
- Theoretical Chemistry
- Browse content in Computer Science
- Artificial Intelligence
- Computer Architecture and Logic Design
- Game Studies
- Human-Computer Interaction
- Mathematical Theory of Computation
- Programming Languages
- Software Engineering
- Systems Analysis and Design
- Virtual Reality
- Browse content in Computing
- Business Applications
- Computer Security
- Computer Games
- Computer Networking and Communications
- Digital Lifestyle
- Graphical and Digital Media Applications
- Operating Systems
- Browse content in Earth Sciences and Geography
- Atmospheric Sciences
- Environmental Geography
- Geology and the Lithosphere
- Maps and Map-making
- Meteorology and Climatology
- Oceanography and Hydrology
- Palaeontology
- Physical Geography and Topography
- Regional Geography
- Soil Science
- Urban Geography
- Browse content in Engineering and Technology
- Agriculture and Farming
- Biological Engineering
- Civil Engineering, Surveying, and Building
- Electronics and Communications Engineering
- Energy Technology
- Engineering (General)
- Environmental Science, Engineering, and Technology
- History of Engineering and Technology
- Mechanical Engineering and Materials
- Technology of Industrial Chemistry
- Transport Technology and Trades
- Browse content in Environmental Science
- Applied Ecology (Environmental Science)
- Conservation of the Environment (Environmental Science)
- Environmental Sustainability
- Environmentalist Thought and Ideology (Environmental Science)
- Management of Land and Natural Resources (Environmental Science)
- Natural Disasters (Environmental Science)
- Nuclear Issues (Environmental Science)
- Pollution and Threats to the Environment (Environmental Science)
- Social Impact of Environmental Issues (Environmental Science)
- History of Science and Technology
- Browse content in Materials Science
- Ceramics and Glasses
- Composite Materials
- Metals, Alloying, and Corrosion
- Nanotechnology
- Browse content in Mathematics
- Applied Mathematics
- Biomathematics and Statistics
- History of Mathematics
- Mathematical Education
- Mathematical Finance
- Mathematical Analysis
- Numerical and Computational Mathematics
- Probability and Statistics
- Pure Mathematics
- Browse content in Neuroscience
- Cognition and Behavioural Neuroscience
- Development of the Nervous System
- Disorders of the Nervous System
- History of Neuroscience
- Invertebrate Neurobiology
- Molecular and Cellular Systems
- Neuroendocrinology and Autonomic Nervous System
- Neuroscientific Techniques
- Sensory and Motor Systems
- Browse content in Physics
- Astronomy and Astrophysics
- Atomic, Molecular, and Optical Physics
- Biological and Medical Physics
- Classical Mechanics
- Computational Physics
- Condensed Matter Physics
- Electromagnetism, Optics, and Acoustics
- History of Physics
- Mathematical and Statistical Physics
- Measurement Science
- Nuclear Physics
- Particles and Fields
- Plasma Physics
- Quantum Physics
- Relativity and Gravitation
- Semiconductor and Mesoscopic Physics
- Browse content in Psychology
- Affective Sciences
- Clinical Psychology
- Cognitive Psychology
- Cognitive Neuroscience
- Criminal and Forensic Psychology
- Developmental Psychology
- Educational Psychology
- Evolutionary Psychology
- Health Psychology
- History and Systems in Psychology
- Music Psychology
- Neuropsychology
- Organizational Psychology
- Psychological Assessment and Testing
- Psychology of Human-Technology Interaction
- Psychology Professional Development and Training
- Research Methods in Psychology
- Social Psychology
- Browse content in Social Sciences
- Browse content in Anthropology
- Anthropology of Religion
- Human Evolution
- Medical Anthropology
- Physical Anthropology
- Regional Anthropology
- Social and Cultural Anthropology
- Theory and Practice of Anthropology
- Browse content in Business and Management
- Business Strategy
- Business Ethics
- Business History
- Business and Government
- Business and Technology
- Business and the Environment
- Comparative Management
- Corporate Governance
- Corporate Social Responsibility
- Entrepreneurship
- Health Management
- Human Resource Management
- Industrial and Employment Relations
- Industry Studies
- Information and Communication Technologies
- International Business
- Knowledge Management
- Management and Management Techniques
- Operations Management
- Organizational Theory and Behaviour
- Pensions and Pension Management
- Public and Nonprofit Management
- Social Issues in Business and Management
- Strategic Management
- Supply Chain Management
- Browse content in Criminology and Criminal Justice
- Criminal Justice
- Criminology
- Forms of Crime
- International and Comparative Criminology
- Youth Violence and Juvenile Justice
- Development Studies
- Browse content in Economics
- Agricultural, Environmental, and Natural Resource Economics
- Asian Economics
- Behavioural Finance
- Behavioural Economics and Neuroeconomics
- Econometrics and Mathematical Economics
- Economic Systems
- Economic History
- Economic Methodology
- Economic Development and Growth
- Financial Markets
- Financial Institutions and Services
- General Economics and Teaching
- Health, Education, and Welfare
- History of Economic Thought
- International Economics
- Labour and Demographic Economics
- Law and Economics
- Macroeconomics and Monetary Economics
- Microeconomics
- Public Economics
- Urban, Rural, and Regional Economics
- Welfare Economics
- Browse content in Education
- Adult Education and Continuous Learning
- Care and Counselling of Students
- Early Childhood and Elementary Education
- Educational Equipment and Technology
- Educational Strategies and Policy
- Higher and Further Education
- Organization and Management of Education
- Philosophy and Theory of Education
- Schools Studies
- Secondary Education
- Teaching of a Specific Subject
- Teaching of Specific Groups and Special Educational Needs
- Teaching Skills and Techniques
- Browse content in Environment
- Applied Ecology (Social Science)
- Climate Change
- Conservation of the Environment (Social Science)
- Environmentalist Thought and Ideology (Social Science)
- Management of Land and Natural Resources (Social Science)
- Natural Disasters (Environment)
- Pollution and Threats to the Environment (Social Science)
- Social Impact of Environmental Issues (Social Science)
- Sustainability
- Browse content in Human Geography
- Cultural Geography
- Economic Geography
- Political Geography
- Browse content in Interdisciplinary Studies
- Communication Studies
- Museums, Libraries, and Information Sciences
- Browse content in Politics
- African Politics
- Asian Politics
- Chinese Politics
- Comparative Politics
- Conflict Politics
- Elections and Electoral Studies
- Environmental Politics
- Ethnic Politics
- European Union
- Foreign Policy
- Gender and Politics
- Human Rights and Politics
- Indian Politics
- International Relations
- International Organization (Politics)
- Irish Politics
- Latin American Politics
- Middle Eastern Politics
- Political Methodology
- Political Communication
- Political Philosophy
- Political Sociology
- Political Behaviour
- Political Economy
- Political Institutions
- Political Theory
- Politics and Law
- Politics of Development
- Public Administration
- Public Policy
- Qualitative Political Methodology
- Quantitative Political Methodology
- Regional Political Studies
- Russian Politics
- Security Studies
- State and Local Government
- UK Politics
- US Politics
- Browse content in Regional and Area Studies
- African Studies
- Asian Studies
- East Asian Studies
- Japanese Studies
- Latin American Studies
- Middle Eastern Studies
- Native American Studies
- Scottish Studies
- Browse content in Research and Information
- Research Methods
- Browse content in Social Work
- Addictions and Substance Misuse
- Adoption and Fostering
- Care of the Elderly
- Child and Adolescent Social Work
- Couple and Family Social Work
- Direct Practice and Clinical Social Work
- Emergency Services
- Human Behaviour and the Social Environment
- International and Global Issues in Social Work
- Mental and Behavioural Health
- Social Justice and Human Rights
- Social Policy and Advocacy
- Social Work and Crime and Justice
- Social Work Macro Practice
- Social Work Practice Settings
- Social Work Research and Evidence-based Practice
- Welfare and Benefit Systems
- Browse content in Sociology
- Childhood Studies
- Community Development
- Comparative and Historical Sociology
- Disability Studies
- Economic Sociology
- Gender and Sexuality
- Gerontology and Ageing
- Health, Illness, and Medicine
- Marriage and the Family
- Migration Studies
- Occupations, Professions, and Work
- Organizations
- Population and Demography
- Race and Ethnicity
- Social Theory
- Social Movements and Social Change
- Social Research and Statistics
- Social Stratification, Inequality, and Mobility
- Sociology of Religion
- Sociology of Education
- Sport and Leisure
- Urban and Rural Studies
- Browse content in Warfare and Defence
- Defence Strategy, Planning, and Research
- Land Forces and Warfare
- Military Administration
- Military Life and Institutions
- Naval Forces and Warfare
- Other Warfare and Defence Issues
- Peace Studies and Conflict Resolution
- Weapons and Equipment

- < Previous
- Next chapter >

1 Aristotle on the Principles of Change in Physics I
- Published: February 2006
- Cite Icon Cite
- Permissions Icon Permissions
This essay is a commentary on Physics I, with special reference to the account of change in chapter 7. It is argued: (i) that Aristotle is mistaken when he attempts in the earlier chapters to present his account as the natural development of various Pre-Socratic views ‘on nature’; (ii) that as a result the main theme of his chapter 5 is a clear error; and (iii) that this raises an important question over how we should understand the apparent claims of chapter 6. All of this leads to the problem: How should we explain his remark at the end of chapter 7 that it is not yet clear whether the underlying thing, the thing that persists through change, is substance ?
Personal account
- Sign in with email/username & password
- Get email alerts
- Save searches
- Purchase content
- Activate your purchase/trial code
- Add your ORCID iD
Institutional access
Sign in with a library card.
- Sign in with username/password
- Recommend to your librarian
- Institutional account management
- Get help with access
Access to content on Oxford Academic is often provided through institutional subscriptions and purchases. If you are a member of an institution with an active account, you may be able to access content in one of the following ways:
IP based access
Typically, access is provided across an institutional network to a range of IP addresses. This authentication occurs automatically, and it is not possible to sign out of an IP authenticated account.
Choose this option to get remote access when outside your institution. Shibboleth/Open Athens technology is used to provide single sign-on between your institution’s website and Oxford Academic.
- Click Sign in through your institution.
- Select your institution from the list provided, which will take you to your institution's website to sign in.
- When on the institution site, please use the credentials provided by your institution. Do not use an Oxford Academic personal account.
- Following successful sign in, you will be returned to Oxford Academic.
If your institution is not listed or you cannot sign in to your institution’s website, please contact your librarian or administrator.
Enter your library card number to sign in. If you cannot sign in, please contact your librarian.
Society Members
Society member access to a journal is achieved in one of the following ways:
Sign in through society site
Many societies offer single sign-on between the society website and Oxford Academic. If you see ‘Sign in through society site’ in the sign in pane within a journal:
- Click Sign in through society site.
- When on the society site, please use the credentials provided by that society. Do not use an Oxford Academic personal account.
If you do not have a society account or have forgotten your username or password, please contact your society.
Sign in using a personal account
Some societies use Oxford Academic personal accounts to provide access to their members. See below.
A personal account can be used to get email alerts, save searches, purchase content, and activate subscriptions.
Some societies use Oxford Academic personal accounts to provide access to their members.
Viewing your signed in accounts
Click the account icon in the top right to:
- View your signed in personal account and access account management features.
- View the institutional accounts that are providing access.
Signed in but can't access content
Oxford Academic is home to a wide variety of products. The institutional subscription may not cover the content that you are trying to access. If you believe you should have access to that content, please contact your librarian.
For librarians and administrators, your personal account also provides access to institutional account management. Here you will find options to view and activate subscriptions, manage institutional settings and access options, access usage statistics, and more.
Our books are available by subscription or purchase to libraries and institutions.
Month: | Total Views: |
---|---|
October 2022 | 23 |
November 2022 | 17 |
December 2022 | 9 |
January 2023 | 4 |
February 2023 | 15 |
March 2023 | 14 |
April 2023 | 17 |
May 2023 | 15 |
June 2023 | 2 |
August 2023 | 4 |
September 2023 | 6 |
October 2023 | 10 |
November 2023 | 9 |
December 2023 | 10 |
January 2024 | 15 |
February 2024 | 13 |
March 2024 | 2 |
April 2024 | 7 |
May 2024 | 2 |
June 2024 | 1 |
July 2024 | 7 |
August 2024 | 2 |
- About Oxford Academic
- Publish journals with us
- University press partners
- What we publish
- New features
- Open access
- Rights and permissions
- Accessibility
- Advertising
- Media enquiries
- Oxford University Press
- Oxford Languages
- University of Oxford
Oxford University Press is a department of the University of Oxford. It furthers the University's objective of excellence in research, scholarship, and education by publishing worldwide
- Copyright © 2024 Oxford University Press
- Cookie settings
- Cookie policy
- Privacy policy
- Legal notice
This Feature Is Available To Subscribers Only
Sign In or Create an Account
This PDF is available to Subscribers Only
For full access to this pdf, sign in to an existing account, or purchase an annual subscription.

- Table of Contents
- Random Entry
- Chronological
- Editorial Information
- About the SEP
- Editorial Board
- How to Cite the SEP
- Special Characters
- Advanced Tools
- Support the SEP
- PDFs for SEP Friends
- Make a Donation
- SEPIA for Libraries
- Entry Contents
Bibliography
Academic tools.
- Friends PDF Preview
- Author and Citation Info
- Back to Top
Experiment in Physics
Physics, and natural science in general, is a reasonable enterprise based on valid experimental evidence, criticism, and rational discussion. It provides us with knowledge of the physical world, and it is experiment that provides the evidence that grounds this knowledge. Experiment plays many roles in science. One of its important roles is to test theories and to provide the basis for scientific knowledge. [ 1 ] It can also call for a new theory, either by showing that an accepted theory is incorrect, or by exhibiting a new phenomenon that is in need of explanation. Experiment can provide hints toward the structure or mathematical form of a theory and it can provide evidence for the existence of the entities involved in our theories. Finally, it may also have a life of its own, independent of theory. Scientists may investigate a phenomenon just because it looks interesting. Such experiments may provide evidence for a future theory to explain. [Examples of these different roles will be presented below.] As we shall see below, a single experiment may play several of these roles at once.
If experiment is to play these important roles in science then we must have good reasons to believe experimental results, for science is a fallible enterprise. Theoretical calculations, experimental results, or the comparison between experiment and theory may all be wrong. Science is more complex than “The scientist proposes, Nature disposes.” It may not always be clear what the scientist is proposing. Theories often need to be articulated and clarified. It also may not be clear how Nature is disposing. Experiments may not always give clear-cut results, and may even disagree for a time.
In what follows, the reader will find an epistemology of experiment, a set of strategies that provides reasonable belief in experimental results. Scientific knowledge can then be reasonably based on these experimental results.
1. Introduction: Epistemology of Experiment
2.1.1 representing and intervening, 2.1.2 experimental strategies, 2.1.3 complexity of experimental practice, 2.2.1 the experimenters’ regress, 2.2.2 communal opportunism and plastic resources, 2.2.3 critical responses, 2.2.4 the dance of agency, 2.2.5 hacking’s ‘the social construction of what’, 2.3 measuring, calibrating, predicting, 2.4 big science physics: theory-ladenness in high energy physics, 3.1 a life of its own, 3.2.1 a crucial experiment: the discovery of parity nonconservation, 3.2.2 a persuasive experiment: the discovery of cp violation, 3.2.3 confirmation after 70 years: the discovery of bose-einstein condensation, 3.3.1 the fall of the fifth force, 3.3.2 right experiment, wrong theory: the stern-gerlach experiment, 3.3.3 sometimes refutation doesn’t work: the double-scattering of electrons, 3.3.4 the failure to detect anomalies, 3.3.5 the ‘look elsewhere’ effect: discovering the higgs boson, 3.4.1 evidence for a new entity: j.j. thomson and the electron, 3.4.2 the articulation of theory: weak interactions, 4. experiment and observation, 5.1 epistemological strategies and the peppered moth experiment, 5.2 the meselson-stahl experiment: “the most beautiful experiment in biology”, 6. computer simulations and experimentation, 7. conclusion, principal works, other suggested reading, other internet resources, related entries.
Epistemology of experiment is a branch of philosophy of science focusing on the diverse roles that experiment plays in science, its various connections to theory, to the understanding and functions of experimental apparatus, and to the structure and culture of the scientific community in the laboratory setting. The epistemological analysis of experiments ranges from highly abstract philosophical arguments with only indirect connection to actual practice, to analysis immersed in reflective case studies. For a long time experiments in physics have been the leading edge of experimental science, pioneering experimental techniques, methods and innovative settings. This is why much of epistemology of experiment has focused on physics.
The 17th century witnessed the first philosophical reflections on the nature of experimentation. This should not be surprising given that experiment was emerging as a central scientific tool at the time. The aim of these reflections was to uncover why nature reveals its hidden aspects to us when we force experimental methods upon it.
Some natural philosophers believed that scientific knowledge was little more than the proper application of observational and experimental techniques on natural phenomena. Francis Bacon went so far as to claim that it was possible to perform what he called a crucial experiment (experimentum crucis), an ideal experiment of sorts that can determine alone which of two rival hypotheses is correct. And even some of the giants of modern science such as Newton subscribed to the view that scientific theories are directly induced from experimental results and observations without the help of untested hypotheses. It is little wonder, then, that many natural philosophers thought that experimental techniques and their proper application should be a primary object of philosophical study of science.
Yet not everybody agreed. Hobbes, for instance pointed out that human reason preceded experimental techniques and their application. He thought that human reasoning reveals to us the natural law, and criticized Boyle’s optimism regarding experimental method’s ability to reveal it (Shapin and Schaffer 1984). Doesn’t human reason guide experimenter’s actions, in the way it leads us to choose data and samples, and the way it allows us to interpret them, after all? If so, we should focus on the philosophical study of reason and theoretical scientific reasoning rather than on the study of experimental techniques and their applications.
This vigorous early debate in many ways anticipated the main points of disagreement in debates to come. Yet the philosophical interest in experimentation almost completely lost its steam at the end of the 19th century and did not recover until fairly late in the 20th century.
During that period philosophers turned much of their attention to the study of the logical structure of scientific theories and its connection to evidence. The tenets of logical positivism influenced this area of investigation — as well as philosophy more generally — at the time. One of these tenets stated that observational and theoretical propositions in science are separable. My readings of the gradation on the scale of a mercury thermometer can be separated from rather complicated theoretical statements concerning heat transfer and the theoretical concept of temperature.
In fact, not only can one separate theory and observation, but the former is considered justified only in light of its correspondence with the latter. The theory of heat transfer is confirmed by propositions originating in the kind of readings I perform on my mercury thermometer. Thus, observational propositions are simply a result of an experiment or a set of observations a scientist performs in order to confirm or refute a theory.
Thomas Kuhn and Paul Feyerabend vigorously criticized this view. They argued that observations and experimental results are already part of a theoretical framework and thus cannot confirm a theory independently. Nor there is a theory-neutral language for capturing observations. Even a simple reading of a mercury thermometer inevitably depends on a theoretically-charged concept of temperature. In short, the evidence is always theory-laden.
Yet neither the proponents of logical positivism nor their critics ever attempted to explain the nature of experimentation that produces all-important observational statements. And the reason for this was very simple: they didn’t think that there was anything interesting to explain. Their views on the relationship between theory and evidence were diametrically opposed, but they all found only the final product of experimentation, namely observational statements, philosophically interesting. As a result, the experimental process itself was set aside in their philosophical study of science. This has gradually changed only with the advent of New Experimentalism, with Ian Hacking’s work at its forefront.
2. Experimental Results
2.1 learning from experiment.
It has been more than three decades since Ian Hacking asked, “Do we see through a microscope?” (Hacking 1981). Hacking’s question really asked how do we come to believe in an experimental result obtained with a complex experimental apparatus? How do we distinguish between a valid result [ 2 ] and an artifact created by that apparatus? If experiment is to play all of the important roles in science mentioned above and to provide the evidential basis for scientific knowledge, then we must have good reasons to believe in those results. Hacking provided an extended answer in the second half of Representing and Intervening (1983). He pointed out that even though an experimental apparatus is laden with, at the very least, the theory of the apparatus, observations remain robust despite changes in the theory of the apparatus or in the theory of the phenomenon. His illustration was the sustained belief in microscope images despite the major change in the theory of the microscope when Abbe pointed out the importance of diffraction in its operation. One reason Hacking gave for this is that in making such observations the experimenters intervened—they manipulated the object under observation. Thus, in looking at a cell through a microscope, one might inject fluid into the cell or stain the specimen. One expects the cell to change shape or color when this is done. Observing the predicted effect strengthens our belief in both the proper operation of the microscope and in the observation. This is true in general. Observing the predicted effect of an intervention strengthens our belief in both the proper operation of the experimental apparatus and in the observations made with it.
Hacking also discussed the strengthening of one’s belief in an observation by independent confirmation. The fact that the same pattern of dots—dense bodies in cells—is seen with “different” microscopes, (e.g. ordinary, polarizing, phase-contrast, fluorescence, interference, electron, acoustic etc.) argues for the validity of the observation. One might question whether “different” is a theory-laden term. After all, it is our theory of light and of the microscope that allows us to consider these microscopes as different from each other. Nevertheless, the argument holds. Hacking correctly argues that it would be a preposterous coincidence if the same pattern of dots were produced in two totally different kinds of physical systems. Different apparatuses have different backgrounds and systematic errors, making the coincidence, if it is an artifact, most unlikely. If it is a correct result, and the instruments are working properly, the coincidence of results is understandable.
Hacking’s answer is correct as far as it goes. It is, however, incomplete. What happens when one can perform the experiment with only one type of apparatus, such as an electron microscope or a radio telescope, or when intervention is either impossible or extremely difficult? Other strategies are needed to validate the observation. [ 3 ] These may include:
- Experimental checks and calibration, in which the experimental apparatus reproduces known phenomena. For example, if we wish to argue that the spectrum of a substance obtained with a new type of spectrometer is correct, we might check that this new spectrometer could reproduce the known Balmer series in hydrogen. If we correctly observe the Balmer Series then we strengthen our belief that the spectrometer is working properly. This also strengthens our belief in the results obtained with that spectrometer. If the check fails then we have good reason to question the results obtained with that apparatus.
- Reproducing artifacts that are known in advance to be present. An example of this comes from experiments to measure the infrared spectra of organic molecules (Randall et al. 1949). It was not always possible to prepare a pure sample of such material. Sometimes the experimenters had to place the substance in an oil paste or in solution. In such cases, one expects to observe the spectrum of the oil or the solvent, superimposed on that of the substance. One can then compare the composite spectrum with the known spectrum of the oil or the solvent. Observation then of this artifact gives confidence in other measurements made with the spectrometer.
- Elimination of plausible sources of error and alternative explanations of the result (the Sherlock Holmes strategy). [ 4 ] Thus, when scientists claimed to have observed electric discharges in the rings of Saturn, they argued for their result by showing that it could not have been caused by defects in the telemetry, interaction with the environment of Saturn, lightning, or dust. The only remaining explanation of their result was that it was due to electric discharges in the rings—there was no other plausible explanation of the observation. (In addition, the same result was observed by both Voyager 1 and Voyager 2. This provided independent confirmation. Often, several epistemological strategies are used in the same experiment.)
- Using the results themselves to argue for their validity. Consider the problem of Galileo’s telescopic observations of the moons of Jupiter. Although one might very well believe that his primitive, early telescope might have produced spurious spots of light, it is extremely implausible that the telescope would create images that they would appear to be a eclipses and other phenomena consistent with the motions of a small planetary system. It would have been even more implausible to believe that the created spots would satisfy Kepler’s Third Law (\(\bfrac{R^3}{T^2} = \) constant). A similar argument was used by Robert Millikan to support his observation of the quantization of electric charge and his measurement of the charge of the electron. Millikan remarked, “The total number of changes which we have observed would be between one and two thousand, and in not one single instance has there been any change which did not represent the advent upon the drop of one definite invariable quantity of electricity or a very small multiple of that quantity ” (Millikan 1911, p. 360). In both of these cases one is arguing that there was no plausible malfunction of the apparatus, or background, that would explain the observations.
- Using an independently well-corroborated theory of the phenomena to explain the results. This was illustrated in the discovery of the \(\ce{W^{\pm}}\), the charged intermediate vector boson required by the Weinberg-Salam unified theory of electroweak interactions. Although these experiments used very complex apparatuses and used other epistemological strategies (for details see Franklin 1986, pp. 170–72). I believe that the agreement of the observations with the theoretical predictions of the particle properties helped to validate the experimental results. In this case the particle candidates were observed in events that contained an electron with high transverse momentum and in which there were no particle jets, just as predicted by the theory. In addition, the measured particle mass of \(81\pm 5\) GeV/c 2 and \(80^{+10}_{-6}\) GeV/c 2 , found in the two experiments (note the independent confirmation also), was in good agreement with the theoretical prediction of \(82\pm 2.4\) GeV/c 2 . It was very improbable that any background effect, which might mimic the presence of the particle, would be in agreement with theory.
- Using an apparatus based on a well-corroborated theory. In this case the support for the theory inspires confidence in the apparatus based on that theory. This is the case with the electron microscope and the radio telescope, whose operations are based on a well-supported theories, although other strategies are also used to validate the observations made with these instruments.
- Using statistical arguments. An interesting example of this arose in the 1960s when the search for new particles and resonances occupied a substantial fraction of the time and effort of those physicists working in experimental high-energy physics. The usual technique was to plot the number of events observed as a function of the invariant mass of the final-state particles and to look for bumps above a smooth background. The usual informal criterion for the presence of a new particle was that it resulted in a three standard-deviation effect above the background, a result that had a probability of 0.27% of occurring in a single bin. This criterion was later changed to four standard deviations, which had a probability of 0.0064% when it was pointed out that the number of graphs plotted each year by high-energy physicists made it rather probable, on statistical grounds, that a three standard-deviation effect would be observed.
These strategies along with Hacking’s intervention and independent confirmation constitute an epistemology of experiment. They provide us with good reasons for belief in experimental results, They do not, however, guarantee that the results are correct. There are many experiments in which these strategies are applied, but whose results are later shown to be incorrect (examples will be presented below). Experiment is fallible. Neither are these strategies exclusive or exhaustive. No single one of them, or fixed combination of them, guarantees the validity of an experimental result. Physicists use as many of the strategies as they can conveniently apply in any given experiment.
In How Experiments End (1987), Peter Galison extended the discussion of experiment to more complex situations. In his histories of the measurements of the gyromagnetic ratio of the electron, the discovery of the muon, and the discovery of weak neutral currents, he considered a series of experiments measuring a single quantity, a set of different experiments culminating in a discovery, and two high- energy physics experiments performed by large groups with complex experimental apparatus.
Galison’s view is that experiments end when the experimenters believe that they have a result that will stand up in court—a result that I believe includes the use of the epistemological strategies discussed earlier. Thus, David Cline, one of the weak neutral-current experimenters remarked, “At present I don’t see how to make these effects [the weak neutral current event candidates] go away” (Galison, 1987, p. 235).
Galison emphasizes that, within a large experimental group, different members of the group may find different pieces of evidence most convincing. Thus, in the Gargamelle weak neutral current experiment, several group members found the single photograph of a neutrino-electron scattering event particularly important, whereas for others the difference in spatial distribution between the observed neutral current candidates and the neutron background was decisive. Galison attributes this, in large part, to differences in experimental traditions, in which scientists develop skill in using certain types of instruments or apparatus. In particle physics, for example, there is the tradition of visual detectors, such as the cloud chamber or the bubble chamber, in contrast to the electronic tradition of Geiger and scintillation counters and spark chambers. Scientists within the visual tradition tend to prefer “golden events” that clearly demonstrate the phenomenon in question, whereas those in the electronic tradition tend to find statistical arguments more persuasive and important than individual events. (For further discussion of this issue see Galison 1997).
Galison points out that major changes in theory and in experimental practice and instruments do not necessarily occur at the same time. This persistence of experimental results provides continuity across these conceptual changes. Thus, the experiments on the gyromagnetic ratio spanned classical electromagnetism, Bohr’s old quantum theory, and the new quantum mechanics of Heisenberg and Schrodinger. Robert Ackermann has offered a similar view in his discussion of scientific instruments.
The advantages of a scientific instrument are that it cannot change theories. Instruments embody theories, to be sure, or we wouldn’t have any grasp of the significance of their operation….Instruments create an invariant relationship between their operations and the world, at least when we abstract from the expertise involved in their correct use. When our theories change, we may conceive of the significance of the instrument and the world with which it is interacting differently, and the datum of an instrument may change in significance, but the datum can nonetheless stay the same, and will typically be expected to do so. An instrument reads 2 when exposed to some phenomenon. After a change in theory, [ 5 ] it will continue to show the same reading, even though we may take the reading to be no longer important, or to tell us something other than what we thought originally (Ackermann 1985, p. 33).
Galison also discusses other aspects of the interaction between experiment and theory. Theory may influence what is considered to be a real effect, demanding explanation, and what is considered background. In his discussion of the discovery of the muon, he argues that the calculation of Oppenheimer and Carlson, which showed that showers were to be expected in the passage of electrons through matter, left the penetrating particles, later shown to be muons, as the unexplained phenomenon. Prior to their work, physicists thought the showering particles were the problem, whereas the penetrating particles seemed to be understood.
The role of theory as an “enabling theory,” (i.e., one that allows calculation or estimation of the size of the expected effect and also the size of expected backgrounds) is also discussed by Galison. (See also (Franklin 1995) and the discussion of the Stern-Gerlach experiment below). Such a theory can help to determine whether an experiment is feasible. Galison also emphasizes that elimination of background that might simulate or mask an effect is central to the experimental enterprise, and not a peripheral activity. In the case of the weak neutral current experiments, the existence of the currents depended crucially on showing that the event candidates could not all be due to neutron background. [ 6 ]
There is also a danger that the design of an experiment may preclude observation of a phenomenon. Galison points out that the original design of one of the neutral current experiments, which included a muon trigger, would not have allowed the observation of neutral currents. In its original form the experiment was designed to observe charged currents, which produce a high energy muon. Neutral currents do not. Therefore, having a muon trigger precluded their observation. Only after the theoretical importance of the search for neutral currents was emphasized to the experimenters was the trigger changed. Changing the design did not, of course, guarantee that neutral currents would be observed.
Galison also shows that the theoretical presuppositions of the experimenters may enter into the decision to end an experiment and report the result. Einstein and de Haas ended their search for systematic errors when their value for the gyromagnetic ratio of the electron, \(g = 1\), agreed with their theoretical model of orbiting electrons. This effect of presuppositions might cause one to be skeptical of both experimental results and their role in theory evaluation. Galison’s history shows, however, that, in this case, the importance of the measurement led to many repetitions of the measurement. This resulted in an agreed-upon result that disagreed with theoretical expectations.
Galison has eventually modified his views. In Image and Logic , an extended study of instrumentation in 20th-century high-energy physics, Galison (1997) has extended his argument that there are two distinct experimental traditions within that field—the visual (or image) tradition and the electronic (or logic) tradition. The image tradition uses detectors such as cloud chambers or bubble chambers, which provide detailed and extensive information about each individual event. The electronic detectors used by the logic tradition, such as geiger counters, scintillation counters, and spark chambers, provide less detailed information about individual events, but detect more events. Galison’s view is that experimenters working in these two traditions form distinct epistemic and linguistic groups that rely on different forms of argument. The visual tradition emphasizes the single “golden” event. “On the image side resides a deep-seated commitment to the ‘golden event’: the single picture of such clarity and distinctness that it commands acceptance.” (Galison, 1997, p. 22) “The golden event was the exemplar of the image tradition: an individual instance so complete and well defined, so ‘manifestly’ free of distortion and background that no further data had to be involved” (p. 23). Because the individual events provided in the logic detectors contained less detailed information than the pictures of the visual tradition, statistical arguments based on large numbers of events were required.
Kent Staley (1999) disagrees. He argues that the two traditions are not as distinct as Galison believes:
I show that discoveries in both traditions have employed the same statistical [I would add “and/or probabilistic”] form of argument, even when basing discovery claims on single, golden events. Where Galison sees an epistemic divide between two communities that can only be bridged by creole- or pidgin-like ‘interlanguage,’ there is in fact a shared commitment to a statistical form of experimental argument. (p. 96).
Staley believes that although there is certainly epistemic continuity within a given tradition, there is also a continuity between the traditions. This does not, I believe, mean that the shared commitment comprises all of the arguments offered in any particular instance, but rather that the same methods are often used by both communities. Galison does not deny that statistical methods are used in the image tradition, but he thinks that they are relatively unimportant. “While statistics could certainly be used within the image tradition, it was by no means necessary for most applications” (Galison, 1997, p. 451). In contrast, Galison believes that arguments in the logic tradition “were inherently and inalienably statistical. Estimation of probable errors and the statistical excess over background is not a side issue in these detectors—it is central to the possibility of any demonstration at all” (p. 451).
Although a detailed discussion of the disagreement between Staley and Galison would take us too far from the subject of this essay, they both agree that arguments are offered for the correctness of experimental results. Their disagreement concerns the nature of those arguments. (For further discussion see Franklin, (2002), pp. 9–17).
2.2 The Case Against Learning From Experiment
H. Collins, A. Pickering, and others, have raised objections to the view that experimental results are accepted on the basis of epistemological arguments. They point out that “a sufficiently determined critic can always find a reason to dispute any alleged ‘result’” (MacKenzie 1989, p. 412). Harry Collins, for example, is well known for his skepticism concerning both experimental results and evidence. He develops an argument that he calls the “experimenters’ regress” (Collins 1985, chapter 4, pp. 79–111): What scientists take to be a correct result is one obtained with a good, that is, properly functioning, experimental apparatus. But a good experimental apparatus is simply one that gives correct results. Collins claims that there are no formal criteria that one can apply to decide whether or not an experimental apparatus is working properly. In particular, he argues that calibrating an experimental apparatus by using a surrogate signal cannot provide an independent reason for considering the apparatus to be reliable.
In Collins’ view the regress is eventually broken by negotiation within the appropriate scientific community, a process driven by factors such as the career, social, and cognitive interests of the scientists, and the perceived utility for future work, but one that is not decided by what we might call epistemological criteria, or reasoned judgment. Thus, Collins concludes that his regress raises serious questions concerning both experimental evidence and its use in the evaluation of scientific hypotheses and theories. Indeed, if no way out of the regress can be found, then he has a point.
Collins strongest candidate for an example of the experimenters’ regress is presented in his history of the early attempts to detect gravitational radiation, or gravity waves. (For more detailed discussion of this episode see (Collins 1985; 1994; Franklin 1994; 1997a) In this case, the physics community was forced to compare J. Weber’s claims that he had observed gravity waves with the reports from six other experiments that failed to detect them. On the one hand, Collins argues that the decision between these conflicting experimental results could not be made on epistemological or methodological grounds—he claims that the six negative experiments could not legitimately be regarded as replications [ 7 ] and hence become less impressive. On the other hand, Weber’s apparatus, precisely because the experiments used a new type of apparatus to try to detect a hitherto unobserved phenomenon, [ 8 ] could not be subjected to standard calibration techniques.
The results presented by Weber’s critics were not only more numerous, but they had also been carefully cross-checked. The groups had exchanged both data and analysis programs and confirmed their results. The critics had also investigated whether or not their analysis procedure, the use of a linear algorithm, could account for their failure to observe Weber’s reported results. They had used Weber’s preferred procedure, a nonlinear algorithm, to analyze their own data, and still found no sign of an effect. They had also calibrated their experimental apparatuses by inserting acoustic pulses of known energy and finding that they could detect a signal. Weber, on the other hand, as well as his critics using his analysis procedure, could not detect such calibration pulses.
There were, in addition, several other serious questions raised about Weber’s analysis procedures. These included an admitted programming error that generated spurious coincidences between Weber’s two detectors, possible selection bias by Weber, Weber’s report of coincidences between two detectors when the data had been taken four hours apart, and whether or not Weber’s experimental apparatus could produce the narrow coincidences claimed.
It seems clear that the critics’ results were far more credible than Weber’s. They had checked their results by independent confirmation, which included the sharing of data and analysis programs. They had also eliminated a plausible source of error, that of the pulses being longer than expected, by analyzing their results using the nonlinear algorithm and by explicitly searching for such long pulses. [ 9 ] They had also calibrated their apparatuses by injecting pulses of known energy and observing the output.
Contrary to Collins, I believe that the scientific community made a reasoned judgment and rejected Weber’s results and accepted those of his critics. Although no formal rules were applied (e.g. if you make four errors, rather than three, your results lack credibility; or if there are five, but not six, conflicting results, your work is still credible) the procedure was reasonable.
Pickering has argued that the reasons for accepting results are the future utility of such results for both theoretical and experimental practice and the agreement of such results with the existing community commitments. In discussing the discovery of weak neutral currents, Pickering states,
Quite simply, particle physicists accepted the existence of the neutral current because they could see how to ply their trade more profitably in a world in which the neutral current was real. (1984b, p. 87) Scientific communities tend to reject data that conflict with group commitments and, obversely, to adjust their experimental techniques to tune in on phenomena consistent with those commitments. (1981, p. 236)
The emphasis on future utility and existing commitments is clear. These two criteria do not necessarily agree. For example, there are episodes in the history of science in which more opportunity for future work is provided by the overthrow of existing theory. (See, for example, the history of the overthrow of parity conservation and of CP symmetry discussed below and in Franklin 1986, Ch. 1, 3.)
Pickering offered a different view of experimental results in late 1980s. In his view the material procedure (including the experimental apparatus itself along with setting it up, running it, and monitoring its operation), the theoretical model of that apparatus, and the theoretical model of the phenomena under investigation are all plastic resources that the investigator brings into relations of mutual support. (Pickering 1987; Pickering 1989). He says:
Achieving such relations of mutual support is, I suggest, the defining characteristic of the successful experiment. (1987, p. 199)
He uses Morpurgo’s search for free quarks, or fractional charges of \(\tfrac{1}{3} e\) or \(\tfrac{2}{3} e\), where \(e\) is the charge of the electron. (See also Gooding 1992.) Morpurgo used a modern Millikan-type apparatus and initially found a continuous distribution of charge values. Following some tinkering with the apparatus, Morpurgo found that if he separated the capacitor plates he obtained only integral values of charge. “After some theoretical analysis, Morpurgo concluded that he now had his apparatus working properly, and reported his failure to find any evidence for fractional charges” (Pickering 1987, p. 197).
Pickering goes on to note that Morpurgo did not tinker with the two competing theories of the phenomena then on offer, those of integral and fractional charge:
The initial source of doubt about the adequacy of the early stages of the experiment was precisely the fact that their findings—continuously distributed charges—were consonant with neither of the phenomenal models which Morpurgo was prepared to countenance. And what motivated the search for a new instrumental model was Morpurgo’s eventual success in producing findings in accordance with one of the phenomenal models he was willing to accept The conclusion of Morpurgo’s first series of experiments, then, and the production of the observation report which they sustained, was marked by bringing into relations of mutual support of the three elements I have discussed: the material form of the apparatus and the two conceptual models, one instrumental and the other phenomenal. Achieving such relations of mutual support is, I suggest, the defining characteristic of the successful experiment. (p. 199)
Pickering has made several important and valid points concerning experiment. Most importantly, he has emphasized that an experimental apparatus is initially rarely capable of producing a valid experimental results and that some adjustment, or tinkering, is required before it does. He has also recognized that both the theory of the apparatus and the theory of the phenomena can enter into the production of a valid experimental result. What one may question, however, is the emphasis he places on these theoretical components. From Millikan onwards, experiments had strongly supported the existence of a fundamental unit of charge and charge quantization. The failure of Morpurgo’s apparatus to produce measurements of integral charge indicated that it was not operating properly and that his theoretical understanding of it was faulty. It was the failure to produce measurements in agreement with what was already known (i.e., the failure of an important experimental check) that caused doubts about Morpurgo’s measurements. This was true regardless of the theoretical models available, or those that Morpurgo was willing to accept. It was only when Morpurgo’s apparatus could reproduce known measurements that it could be trusted and used to search for fractional charge. To be sure, Pickering has allowed a role for the natural world in the production of the experimental result, but it does not seem to be decisive.
Ackermann has offered a modification of Pickering’s view. He suggests that the experimental apparatus itself is a less plastic resource then either the theoretical model of the apparatus or that of the phenomenon.
To repeat, changes in \(A\) [the apparatus] can often be seen (in real time, without waiting for accommodation by \(B\) [the theoretical model of the apparatus]) as improvements, whereas ‘improvements’ in \(B\) don’t begin to count unless \(A\) is actually altered and realizes the improvements conjectured. It’s conceivable that this small asymmetry can account, ultimately, for large scale directions of scientific progress and for the objectivity and rationality of those directions. (Ackermann 1991, p. 456)
Hacking (1992) has also offered a more complex version of Pickering’s later view. He suggests that the results of mature laboratory science achieve stability and are self-vindicating when the elements of laboratory science are brought into mutual consistency and support. These are (1) ideas: questions, background knowledge, systematic theory, topical hypotheses, and modeling of the apparatus; (2) things: target, source of modification, detectors, tools, and data generators; and (3) marks and the manipulation of marks: data, data assessment, data reduction, data analysis, and interpretation.
Stable laboratory science arises when theories and laboratory equipment evolve in such a way that they match each other and are mutually self-vindicating. (1992, p. 56) We invent devices that produce data and isolate or create phenomena, and a network of different levels of theory is true to these phenomena. Conversely we may in the end count them only as phenomena only when the data can be interpreted by theory. (pp. 57–8)
One might ask whether such mutual adjustment between theory and experimental results can always be achieved? What happens when an experimental result is produced by an apparatus on which several of the epistemological strategies, discussed earlier, have been successfully applied, and the result is in disagreement with our theory of the phenomenon? Accepted theories can be refuted. Several examples will be presented below.
Hacking himself worries about what happens when a laboratory science that is true to the phenomena generated in the laboratory, thanks to mutual adjustment and self-vindication, is successfully applied to the world outside the laboratory. Does this argue for the truth of the science. In Hacking’s view it does not. If laboratory science does produce happy effects in the “untamed world,… it is not the truth of anything that causes or explains the happy effects” (1992, p. 60).
Pickering offered yet another, a somewhat revised account of science. “My basic image of science is a performative one, in which the performances the doings of human and material agency come to the fore. Scientists are human agents in a field of material agency which they struggle to capture in machines (Pickering, 1995, p. 21).” He then discusses the complex interaction between human and material agency, which I interpret as the interaction between experimenters, their apparatus, and the natural world.
The dance of agency, seen asymmetrically from the human end, thus takes the form of a dialectic of resistance and accommodations, where resistance denotes the failure to achieve an intended capture of agency in practice, and accommodation an active human strategy of response to resistance, which can include revisions to goals and intentions as well as to the material form of the machine in question and to the human frame of gestures and social relations that surround it (p. 22).“
Pickering’s idea of resistance is illustrated by Morpurgo’s observation of continuous, rather than integral or fractional, electrical charge, which did not agree with his expectations. Morpurgo’s accommodation consisted of changing his experimental apparatus by using a larger separation between his plates, and also by modifying his theoretical account of the apparatus. That being done, integral charges were observed and the result stabilized by the mutual agreement of the apparatus, the theory of the apparatus, and the theory of the phenomenon. Pickering notes that ”the outcomes depend on how the world is (p. 182).“ ”In this way, then, how the material world is leaks into and infects our representations of it in a nontrivial and consequential fashion. My analysis thus displays an intimate and responsive engagement between scientific knowledge and the material world that is integral to scientific practice (p. 183).“
Nevertheless there is something confusing about Pickering’s invocation of the natural world. Although Pickering acknowledges the importance of the natural world, his use of the term ”infects“ seems to indicate that he isn’t entirely happy with this. Nor does the natural world seem to have much efficacy. It never seems to be decisive in any of Pickering’s case studies. Recall that he argued that physicists accepted the existence of weak neutral currents because ”they could ply their trade more profitably in a world in which the neutral current was real.“ In his account, Morpurgo’s observation of continuous charge is important only because it disagrees with his theoretical models of the phenomenon. The fact that it disagreed with numerous previous observations of integral charge doesn’t seem to matter. This is further illustrated by Pickering’s discussion of the conflict between Morpurgo and Fairbank. As we have seen, Morpurgo reported that he did not observe fractional electrical charges. On the other hand, in the late 1970s and early 1980s, Fairbank and his collaborators published a series of papers in which they claimed to have observed fractional charges (See, for example, LaRue, Phillips et al. 1981 ). Faced with this discord Pickering concludes,
In Chapter 3, I traced out Morpurgo’s route to his findings in terms of the particular vectors of cultural extension that he pursued, the particular resistances and accommodations thus precipitated, and the particular interactive stabilizations he achieved. The same could be done, I am sure, in respect of Fairbank. And these tracings are all that needs to said about their divergence. It just happened that the contingencies of resistance and accommodation worked out differently in the two instances. Differences like these are, I think, continually bubbling up in practice, without any special causes behind them (pp. 211–212).
The natural world seems to have disappeared from Pickering’s account. There is a real question here as to whether or not fractional charges exist in nature. The conclusions reached by Fairbank and by Morpurgo about their existence cannot both be correct. It seems insufficient to merely state, as Pickering does, that Fairbank and Morpurgo achieved their individual stabilizations and to leave the conflict unresolved. (Pickering does comment that one could follow the subsequent history and see how the conflict was resolved, and he does give some brief statements about it, but its resolution is not important for him). At the very least one should consider the actions of the scientific community. Scientific knowledge is not determined individually, but communally. Pickering seems to acknowledge this. ”One might, therefore, want to set up a metric and say that items of scientific knowledge are more or less objective depending on the extent to which they are threaded into the rest of scientific culture, socially stabilized over time, and so on. I can see nothing wrong with thinking this way…. (p. 196).“ The fact that Fairbank believed in the existence of fractional electrical charges, or that Weber strongly believed that he had observed gravity waves, does not make them right. These are questions about the natural world that can be resolved. Either fractional charges and gravity waves exist or they don’t, or to be more cautious we might say that we have good reasons to support our claims about their existence, or we do not.
Another issue neglected by Pickering is the question of whether a particular mutual adjustment of theory, of the apparatus or the phenomenon, and the experimental apparatus and evidence is justified. Pickering seems to believe that any such adjustment that provides stabilization, either for an individual or for the community, is acceptable. Others disagree. They note that experimenters sometimes exclude data and engage in selective analysis procedures in producing experimental results. These practices are, at the very least, questionable as is the use of the results produced by such practices in science. There are, in fact, procedures in the normal practice of science that provide safeguards against them. (For details see Franklin, 2002, Section 1).
The difference in attitudes toward the resolution of discord is one of the important distinctions between Pickering’s and Franklin’s view of science. Franklin remarks that it is insufficient simply to say that the resolution is socially stabilized. The important question is how that resolution was achieved and what were the reasons offered for that resolution. If we are faced with discordant experimental results and both experimenters have offered reasonable arguments for their correctness, then clearly more work is needed. It seems reasonable, in such cases, for the physics community to search for an error in one, or both, of the experiments.
Pickering discusses yet another difference between his view and that of Franklin. Pickering sees traditional philosophy of science as regarding objectivity ”as stemming from a peculiar kind of mental hygiene or policing of thought. This police function relates specifically to theory choice in science, which,… is usually discussed in terms of the rational rules or methods responsible for closure in theoretical debate (p. 197).“ He goes on to remark that,
The most action in recent methodological thought has centered on attempts like Allan Franklin’s to extend the methodological approach to experiments by setting up a set of rules for their proper performance. Franklin thus seeks to extend classical discussions of objectivity to the empirical base of science (a topic hitherto neglected in the philosophical tradition but one that, of course the mangle [Pickering’s view] also addresses). For an argument between myself and Franklin on the same lines as that laid out below, see (Franklin 1990, Chapter 8; Franklin 1991); and (Pickering 1991); and for commentaries related to that debate, (Ackermann 1991) and (Lynch 1991) (p. 197).”
For further discussion see Franklin 1993b. Although Franklin’s epistemology of experiment is designed to offer good reasons for belief in experimental results, they are not a set of rules. Franklin regards them as a set of strategies, from which physicists choose, in order to argue for the correctness of their results. As noted above, the strategies offered are neither exclusive or exhaustive.
There is another point of disagreement between Pickering and Franklin. Pickering claims to be dealing with the practice of science, and yet he excludes certain practices from his discussions. One scientific practice is the application of the epistemological strategies outlined above to argue for the correctness of an experimental results. In fact, one of the essential features of an experimental paper is the presentation of such arguments. Writing such papers, a performative act, is also a scientific practice and it would seem reasonable to examine both the structure and content of those papers.
Ian Hacking (1999, chapter 3) provided an incisive and interesting discussion of the issues that divide the constructivists (Collins, Pickering, etc.) from the rationalists (Stuewer, Franklin, Buchwald, etc.). He sets out three sticking points between the two views: 1) contingency, 2) nominalism, and 3) external explanations of stability.
Contingency is the idea that science is not predetermined, that it could have developed in any one of several successful ways. This is the view adopted by constructivists. Hacking illustrates this with Pickering’s account of high-energy physics during the 1970s during which the quark model came to dominate. (See Pickering 1984a).
The constructionist maintains a contingency thesis. In the case of physics, (a) physics theoretical, experimental, material) could have developed in, for example, a nonquarky way, and, by the detailed standards that would have evolved with this alternative physics, could have been as successful as recent physics has been by its detailed standards. Moreover, (b) there is no sense in which this imagined physics would be equivalent to present physics. The physicist denies that. (Hacking 1999, pp. 78–79). To sum up Pickering’s doctrine: there could have been a research program as successful (“progressive”) as that of high-energy physics in the 1970s, but with different theories, phenomenology, schematic descriptions of apparatus, and apparatus, and with a different, and progressive, series of robust fits between these ingredients. Moreover and this is something badly in need of clarification the “different” physics would not have been equivalent to present physics. Not logically incompatible with, just different. The constructionist about (the idea) of quarks thus claims that the upshot of this process of accommodation and resistance is not fully predetermined. Laboratory work requires that we get a robust fit between apparatus, beliefs about the apparatus, interpretations and analyses of data, and theories. Before a robust fit has been achieved, it is not determined what that fit will be. Not determined by how the world is, not determined by technology now in existence, not determined by the social practices of scientists, not determined by interests or networks, not determined by genius, not determined by anything (pp. 72–73, emphasis added).
Much depends here on what Hacking means by “determined.” If he means entailed then one must agree with him. It is doubtful that the world, or more properly, what we can learn about it, entails a unique theory. If not, as seems more plausible, he means that the way the world is places no restrictions on that successful science, then the rationalists disagree strongly. They want to argue that the way the world is restricts the kinds of theories that will fit the phenomena, the kinds of apparatus we can build, and the results we can obtain with such apparatuses. To think otherwise seems silly. Consider a homey example. It seems highly unlikely that someone can come up with a successful theory in which objects whose density is greater than that of air fall upwards. This is not a caricature of the view Hacking describes. Describing Pickering’s view, he states, “Physics did not need to take a route that involved Maxwell’s Equations, the Second Law of Thermodynamics, or the present values of the velocity of light (p. 70).” Although one may have some sympathy for this view as regards Maxwell’s Equations or the Second Law of Thermodynamics, one may not agree about the value of the speed of light. That is determined by the way the world is. Any successful theory of light must give that value for its speed.
At the other extreme are the “inevitablists,” among whom Hacking classifies most scientists. He cites Sheldon Glashow, a Nobel Prize winner, “Any intelligent alien anywhere would have come upon the same logical system as we have to explain the structure of protons and the nature of supernovae (Glashow 1992, p. 28).”
Another difference between Pickering and Franklin on contingency concerns the question of not whether an alternative is possible, but rather whether there are reasons why that alternative should be pursued. Pickering seems to identify can with ought .
In the late 1970s there was a disagreement between the results of low-energy experiments on atomic parity violation (the violation of left-right symmetry) performed at the University of Washington and at Oxford University and the result of a high-energy experiment on the scattering of polarized electrons from deuterium (the SLAC E122 experiment). The atomic-parity violation experiments failed to observe the parity-violating effects predicted by the Weinberg- Salam (W-S) unified theory of electroweak interactions, whereas the SLAC experiment observed the predicted effect. These early atomic physics results were quite uncertain in themselves and that uncertainty was increased by positive results obtained in similar experiments at Berkeley and Novosibirsk. At the time the theory had other evidential support, but was not universally accepted. Pickering and Franklin are in agreement that the W-S theory was accepted on the basis of the SLAC E122 result. They differ dramatically in their discussions of the experiments. Their difference on contingency concerns a particular theoretical alternative that was proposed at the time to explain the discrepancy between the experimental results.
Pickering asked why a theorist might not have attempted to find a variant of electroweak gauge theory that might have reconciled the Washington-Oxford atomic parity results with the positive E122 result. (What such a theorist was supposed to do with the supportive atomic parity results later provided by experiments at Berkeley and at Novosibirsk is never mentioned). “But though it is true that E122 analysed their data in a way that displayed the improbability [the probability of the fit to the hybrid model was 6 × 10 −4 ] of a particular class of variant gauge theories, the so-called ‘hybrid models,’ I do not believe that it would have been impossible to devise yet more variants” (Pickering 1991, p. 462). Pickering notes that open-ended recipes for constructing such variants had been written down as early as 1972 (p. 467). It would have been possible to do so, but one may ask whether or not a scientist might have wished to do so. If the scientist agreed with Franklin’s view that the SLAC E122 experiment provided considerable evidential weight in support of the W-S theory and that a set of conflicting and uncertain results from atomic parity-violation experiments gave an equivocal answer on that support, what reason would they have had to invent an alternative?
This is not to suggest that scientists do not, or should not, engage in speculation, but rather that there was no necessity to do so in this case. Theorists often do propose alternatives to existing, well-confirmed theories.
Constructivist case studies always seem to result in the support of existing, accepted theory (Pickering 1984a; 1984b; 1991; Collins 1985; Collins and Pinch 1993). One criticism implied in such cases is that alternatives are not considered, that the hypothesis space of acceptable alternatives is either very small or empty. One may seriously question this. Thus, when the experiment of Christenson et al . (1964) detected \(\ce{K2^0}\) decay into two pions, which seemed to show that CP symmetry (combined particle-antiparticle and space inversion symmetry) was violated, no fewer than 10 alternatives were offered. These included (1) the cosmological model resulting from the local dysymmetry of matter and antimatter, (2) external fields, (3) the decay of the \(\ce{K2^0}\) into a \(\ce{K1^0}\) with the subsequent decay of the \(\ce{K1^0}\)into two pions, which was allowed by the symmetry, (4) the emission of another neutral particle, “the paritino,” in the \(\ce{K2^0}\) decay, similar to the emission of the neutrino in beta decay, (5) that one of the pions emitted in the decay was in fact a “spion,” a pion with spin one rather than zero, (6) that the decay was due to another neutral particle, the L, produced coherently with the \(\ce{K^0}\), (7) the existence of a “shadow” universe, which interacted with out universe only through the weak interactions, and that the decay seen was the decay of the “shadow \(\ce{K2^0}\),” (8) the failure of the exponential decay law, 9) the failure of the principle of superposition in quantum mechanics, and 10) that the decay pions were not bosons.
As one can see, the limits placed on alternatives were not very stringent. By the end of 1967, all of the alternatives had been tested and found wanting, leaving CP symmetry unprotected. Here the differing judgments of the scientific community about what was worth proposing and pursuing led to a wide variety of alternatives being tested.
Hacking’s second sticking point is nominalism, or name-ism. He notes that in its most extreme form nominalism denies that there is anything in common or peculiar to objects selected by a name, such as “Douglas fir” other than that they are called Douglas fir. Opponents contend that good names, or good accounts of nature, tell us something correct about the world. This is related to the realism-antirealism debate concerning the status of unobservable entities that has plagued philosophers for millennia. For example Bas van Fraassen (1980), an antirealist, holds that we have no grounds for belief in unobservable entities such as the electron and that accepting theories about the electron means only that we believe that the things the theory says about observables is true. A realist claims that electrons really exist and that as, for example, Wilfred Sellars remarked, “to have good reason for holding a theory is ipso facto to have good reason for holding that the entities postulated by the theory exist (Sellars 1962, p. 97).” In Hacking’s view a scientific nominalist is more radical than an antirealist and is just as skeptical about fir trees as they are about electrons. A nominalist further believes that the structures we conceive of are properties of our representations of the world and not of the world itself. Hacking refers to opponents of that view as inherent structuralists.
Hacking also remarks that this point is related to the question of “scientific facts.” Thus, constructivists Latour and Woolgar originally entitled their book Laboratory Life: The Social Construction of Scientific Facts (1979). Andrew Pickering entitled his history of the quark model Constructing Quarks (Pickering 1984a). Physicists argue that this demeans their work. Steven Weinberg, a realist and a physicist, criticized Pickering’s title by noting that no mountaineer would ever name a book Constructing Everest . For Weinberg, quarks and Mount Everest have the same ontological status. They are both facts about the world. Hacking argues that constructivists do not, despite appearances, believe that facts do not exist, or that there is no such thing as reality. He cites Latour and Woolgar “that ‘out-there-ness’ is a consequence of scientific work rather than its cause (Latour and Woolgar 1986, p. 180).” Hacking reasonably concludes that,
Latour and Woolgar were surely right. We should not explain why some people believe that \(p\) by saying that \(p\) is true, or corresponds to a fact, or the facts. For example: someone believes that the universe began with what for brevity we call a big bang. A host of reasons now supports this belief. But after you have listed all the reasons, you should not add, as if it were an additional reason for believing in the big bang, ‘and it is true that the universe began with a big bang.’ Or ‘and it is a fact.’This observation has nothing peculiarly to do with social construction. It could equally have been advanced by an old-fashioned philosopher of language. It is a remark about the grammar of the verb ‘to explain’ (Hacking 1999, pp. 80–81).
One might add, however, that the reasons Hacking cites as supporting that belief are given to us by valid experimental evidence and not by the social and personal interests of scientists. Latour and Woolgar might not agree. Franklin argues that we have good reasons to believe in facts, and in the entities involved in our theories, always remembering, of course, that science is fallible.
Hacking’s third sticking point is the external explanations of stability.
The constructionist holds that explanations for the stability of scientific belief involve, at least in part, elements that are external to the content of science. These elements typically include social factors, interests, networks, or however they be described. Opponents hold that whatever be the context of discovery, the explanation of stability is internal to the science itself (Hacking 1999, p. 92). Rationalists think that most science proceeds as it does in the light of good reasons produced by research. Some bodies of knowledge become stable because of the wealth of good theoretical and experimental reasons that can be adduced for them. Constructivists think that the reasons are not decisive for the course of science. Nelson (1994) concludes that this issue will never be decided. Rationalists, at least retrospectively, can always adduce reasons that satisfy them. Constructivists, with equal ingenuity, can always find to their own satisfaction an openness where the upshot of research is settled by something other than reason. Something external. That is one way of saying we have found an irresoluble “sticking point” (pp. 91–92)
Thus, there is a rather severe disagreement on the reasons for the acceptance of experimental results. For some, like Staley, Galison and Franklin, it is because of epistemological arguments. For others, like Pickering, the reasons are utility for future practice and agreement with existing theoretical commitments. Although the history of science shows that the overthrow of a well-accepted theory leads to an enormous amount of theoretical and experimental work, proponents of this view seem to accept it as unproblematical that it is always agreement with existing theory that has more future utility. Hacking and Pickering also suggest that experimental results are accepted on the basis of the mutual adjustment of elements which includes the theory of the phenomenon.
Nevertheless, everyone seems to agree that a consensus does arise on experimental results.
We have encountered (Section 2.2.1) Franklin’s definition of calibration as the use of a surrogate signal that has been established independently of the apparatus being calibrated, or as the reproduction of independently (on an independent apparatus) well established phenomena. He used this account in order to argue against Collins’s view of the viciousness of the experimenter’s regress. Yet other accounts of calibration explaining the process of measurement evoke similar worries.
E. Tal defines calibration as “the activity of establishing a correlation between indications of a measuring instrument and quantity values associated with a measurement standard” (Tal 2017a, 243). Mere “instrument indications” (Tal 2017a) become “measurement outcomes” when interpreted in a larger context of theoretical background and calibration of the measuring instrument. Yet Boyd (2021, 43) worries that this leaves the measurement open to the vicious regress akin to the experimenter’s regress H. Collins has argued for (see Section 2.2.1), since the experimenters do not “have access to true values of the measurand [i.e., whatever is measured] to use as standards of calibration”.
The regress may be blocked by comparisons across measuring outcomes performed in differently modeled measurements, i.e. across different measurement contexts (the context being constituted by intricacies of the operational and theoretical background of apparata). (Tal 2017a, 239) These different contexts are idealized and then compared in order to establish whether they cohere to the predictions concerning the measurand. Thus, calibration is “the activity of modeling different processes and testing the consequences of such models for mutual compatibility” (Tal 2017a, 246). An act of calibration is effectively the comparison of idealized models across measuring processes, where an act of measurement becomes justified by the models’ mutual coherence.
Boyd (2021, 46) states that idealizations of measurement processes cannot provide the foundation for objective measurement since idealizations strip the context of its key details. In fact, the measurement has an epistemic utility by virtue of the context-sensitive details. The measurement outcomes gain epistemic value only as “enriched evidence”, i.e., in the wider empirical and theoretical context.
Moreover, Tal’s view conflates prediction and calibration (Boyd 2021, 48) taking us back to the viciousness of the experimenter’s regress. Thus, “Calibration was supposed to disrupt the regress because an instrument could be judged to be working correctly in virtue of something other than success at its principal aim” (Boyd 2021, 48). Calibration has to provide a point outside the web of cohering predictions if it is to break the regress, e.g. by the subtle processing of instrument indications.
Progressive coherentism’s attempted way out of the vicious regress, as proposed by Hasok Chang (2004, 2007), points to a historical trajectory of the development of measuring procedures and calibration. The web of cohering measuring process is dynamic: it unfolds in time as a spiral. And the measurement process that initially aims at prediction eventually becomes a result used for calibrating processes. Yet, such spiraling progress may be just an elaborate dynamic coherence web of Collins’ type where inter-subjective agreement is the foundation, if other non-empirical virtues such as “creative achievement” play as important role as the empirical ones as claimed by Chang (2007). If so, calibration does not offer an independent leverage to the measurement outcomes after all.
Perović (2017) analyzes in-situ calibration procedures in the Large Hadron Collider pointing out that calibration serves as an epistemic leverage of Franklin’s type during the commissioning phase of the experimental apparatus. But various calibration procedures continue all along and gradually feed into the measurement itself during the entire measurement process. Boyd (2021) further explores commissioning procedures and the gradual transition from “engineering data“ to “science data”, where criteria of prediction significantly change. The apparatus initially relies on the existing well-known data but then coherence with other apparata that Tal insists on becomes increasingly less significant in justifying the measurement outcomes.
Authors like Thomas Kuhn and Paul Feyerabend put forward the view that evidence does not confirm or refute a scientific theory since it is laden by it. Evidence is not a set of observational sentences autonomous from theoretical ones, as logical positivists believed. Each new theory or a theoretical paradigm, as Kuhn labeled larger theoretical frameworks, produces, as it were, evidence anew.
Thus, theoretical concepts infect the entire experimental process from the stage of design and preparation to the production and analysis of data. A simple example that is supposed to convincingly illustrate this view are measurements of temperature with a mercury thermometer one uses in order to test whether objects expand when their temperature increases. Note that in such a case one tests the hypothesis by relying on the very assumption that the expansion of mercury indicates increase in temperature.
There may be a fairly simple way out of the vicious circle in which theory and experiment are caught in this particular case of theory-ladenness. It may suffice to calibrate the mercury thermometer with a constant volume gas thermometer, for example, where its use does not rely on the tested hypothesis but on the proportionality of the pressure of the gas and its absolute temperature (Franklin et al. 1989).
Although most experiments are far more complex than this toy example, one could certainly approach the view that experimental results are theory-laden on a case-by-case basis. Yet there may be a more general problem with the view.
Bogen and Woodward (1988) argued that debate on the relationship between theory and observation overlooks a key ingredient in the production of experimental evidence, namely the experimental phenomena. The experimentalists distill experimental phenomena from raw experimental data (e.g. electronic or digital tracks in particle colliders) using various tools of statistical analysis. Thus, identification of an experimental phenomenon as significant (e.g. a peak at a particular energy of colliding beams) is free of the theory that the experiment may be designed to test (e.g. the prediction of a particular particle). Only when significant phenomenon has been identified can a stage of data analysis begin in which the phenomenon is deemed to either support or refute a theory. Thus, the theory-ladenness of evidence thesis fails at least in some experiments in physics.
The authors substantiate their argument in part through an analysis of experiments that led to a breakthrough discovery of weak neutral currents. It is a type of force produced by so-called bosons — short-lived particles responsible for energy transfer between other particles such as hadrons and leptons. The relevant peaks were recognized as significant via statistical analysis of data, and later on interpreted as evidence for the existence of the bosons.
This view and the case study have been challenged by Schindler (2011). He argues that the tested theory was critical in the assessment of the reliability of data in the experiments with weak neutral currents. He also points out that, on occasion, experimental data can even be ignored if they are deemed irrelevant from a theoretical perspective that physicists find particularly compelling. This was the case in experiments with so-called zebra pattern magnetic anomalies on the ocean floor. The readings of new apparatuses used to scan the ocean floor produced intriguing signals. Yet the researchers could not interpret these signals meaningfully or satisfyingly distinguish them from noise unless they relied on some theoretical account of both the structure of the ocean floor and the earth’s magnetic field.
Karaca (2013) points out that a crude theory-observation distinction is particularly unhelpful in understanding high-energy physics experiments. It fails to capture the complexity of relevant theoretical structures and their relation to experimental data. Theoretical structures can be composed of background, model, and phenomenological theories. Background theories are very general theories (e.g. quantum field theory or quantum electrodynamics) that define the general properties of physical particles and their interactions. Models are specific instances of background theories that define particular particles and their properties. While phenomenological theories develop testable predictions based on these models.
Now, each of these theoretical segments stands in a different relationship to experimental data—the experiments can be laden by a different segment to a different extent. This requires a nuanced categorization of theory-ladeness, from weak to strong.
Thus, an experimental apparatus can be designed to test a very specific theoretical model. UA1 and UA2 detectors at CERN’s Super Proton Synchrotron were designed to detect particles only in a very specific energy regime in which W and Z bosons of the Standard Model were expected to exist.
In contrast, exploratory experiments approach phenomena without relying on a particular theoretical model. Thus, sometimes a theoretical framework for an experiment consists of phenomenological theory alone. Karaca argues that experiments with deep-inelastic electron-proton scattering in the late 1960s and early 1970s are example of such weakly theory-laden experiments. The application of merely phenomenological parameters in the experiment resulted in the very important discovery of the composite rather than point-like structure of hadrons (protons and neutrons), or the so-called scaling law. And this eventually led to a successful theoretical model of the composition of hadrons, namely quantum chromodynamics, or the quark-model of strong interactions.

3. The Roles of Experiment
Although experiment often takes its importance from its relation to theory, Hacking pointed out that it often has a life of its own, independent of theory. He notes the pristine observations of Carolyn Herschel’s discovery of comets, William Herschel’s work on “radiant heat,” and Davy’s observation of the gas emitted by algae and the flaring of a taper in that gas. In none of these cases did the experimenter have any theory of the phenomenon under investigation. One may also note the nineteenth century measurements of atomic spectra and the work on the masses and properties on elementary particles during the 1960s. Both of these sequences were conducted without any guidance from theory.
In deciding what experimental investigation to pursue, scientists may very well be influenced by the equipment available and their own ability to use that equipment (McKinney 1992). Thus, when the Mann-O’Neill collaboration was doing high energy physics experiments at the Princeton-Pennsylvania Accelerator during the late 1960s, the sequence of experiments was (1) measurement of the \(\ce{K+}\) decay rates, (2) measurement of the \(\ce{K+_{e 3}}\) branching ratio and decay spectrum, (3) measurement of the \(\ce{K+_{e 2}}\) branching ratio, and (4) measurement of the form factor in \(\ce{K+_{e 3}}\) decay. These experiments were performed with basically the same experimental apparatus, but with relatively minor modifications for each particular experiment. By the end of the sequence the experimenters had become quite expert in the use of the apparatus and knowledgeable about the backgrounds and experimental problems. This allowed the group to successfully perform the technically more difficult experiments later in the sequence. We might refer to this as “instrumental loyalty” and the “recycling of expertise” (Franklin 1997b). This meshes nicely with Galison’s view of experimental traditions. Scientists, both theorists and experimentalists, tend to pursue experiments and problems in which their training and expertise can be used.
Hacking also remarks on the “noteworthy observations” on Iceland Spar by Bartholin, on diffraction by Hooke and Grimaldi, and on the dispersion of light by Newton. “Now of course Bartholin, Grimaldi, Hooke, and Newton were not mindless empiricists without an ‘idea’ in their heads. They saw what they saw because they were curious, inquisitive, reflective people. They were attempting to form theories. But in all these cases it is clear that the observations preceded any formulation of theory” (Hacking 1983, p. 156). In all of these cases we may say that these were observations waiting for, or perhaps even calling for, a theory. The discovery of any unexpected phenomenon calls for a theoretical explanation.
3.2 Confirmation and Refutation
Nevertheless several of the important roles of experiment involve its relation to theory. Experiment may confirm a theory, refute a theory, or give hints to the mathematical structure of a theory.
Let us consider first an episode in which the relation between theory and experiment was clear and straightforward. This was a “crucial” experiment, one that decided unequivocally between two competing theories, or classes of theory. The episode was that of the discovery that parity, mirror-reflection symmetry or left-right symmetry, is not conserved in the weak interactions. (For details of this episode see Franklin (1986, Ch. 1) and Appendix 1 ). Experiments showed that in the beta decay of nuclei the number of electrons emitted in the same direction as the nuclear spin was different from the number emitted opposite to the spin direction. This was a clear demonstration of parity violation in the weak interactions.
After the discovery of parity and charge conjugation nonconservation, and following a suggestion by Landau, physicists considered CP (combined parity and particle-antiparticle symmetry), which was still conserved in the experiments, as the appropriate symmetry. One consequence of this scheme, if CP were conserved, was that the \(\ce{K1^0}\) meson could decay into two pions, whereas the \(\ce{K2^0}\) meson could not. [ 10 ] Thus, observation of the decay of \(\ce{K2^0}\) into two pions would indicate CP violation. The decay was observed by a group at Princeton University. Although several alternative explanations were offered, experiments eliminated each of the alternatives leaving only CP violation as an explanation of the experimental result. (For details of this episode see Franklin (1986, Ch. 3) and Appendix 2 .)
In both of the episodes discussed previously, those of parity nonconservation and of CP violation, we saw a decision between two competing classes of theories. This episode, the discovery of Bose-Einstein condensation (BEC), illustrates the confirmation of a specific theoretical prediction 70 years after the theoretical prediction was first made. Bose (1924) and Einstein (1924; 1925) predicted that a gas of noninteracting bosonic atoms will, below a certain temperature, suddenly develop a macroscopic population in the lowest energy quantum state. [ 11 ] (For details of this episode see Appendix 3 .)
3.3 Complications
In the three episodes discussed in the previous section, the relation between experiment and theory was clear. The experiments gave unequivocal results and there was no ambiguity about what theory was predicting. None of the conclusions reached has since been questioned. Parity and CP symmetry are violated in the weak interactions and Bose-Einstein condensation is an accepted phenomenon. In the practice of science things are often more complex. Experimental results may be in conflict, or may even be incorrect. Theoretical calculations may also be in error or a correct theory may be incorrectly applied. There are even cases in which both experiment and theory are wrong. As noted earlier, science is fallible. In this section I will discuss several episodes which illustrate these complexities.
The episode of the fifth force is the case of a refutation of an hypothesis, but only after a disagreement between experimental results was resolved. The “Fifth Force” was a proposed modification of Newton’s Law of Universal Gravitation. The initial experiments gave conflicting results: one supported the existence of the Fifth Force whereas the other argued against it. After numerous repetitions of the experiment, the discord was resolved and a consensus reached that the Fifth Force did not exist. (For details of this episode see Appendix 4 .)
The Stern-Gerlach experiment was regarded as crucial at the time it was performed, but, in fact, wasn’t. [ 12 ] In the view of the physics community it decided the issue between two theories, refuting one and supporting the other. In the light of later work, however, the refutation stood, but the confirmation was questionable. In fact, the experimental result posed problems for the theory it had seemingly confirmed. A new theory was proposed and although the Stern-Gerlach result initially also posed problems for the new theory, after a modification of that new theory, the result confirmed it. In a sense, it was crucial after all. It just took some time.
The Stern-Gerlach experiment provides evidence for the existence of electron spin. These experimental results were first published in 1922, although the idea of electron spin wasn’t proposed by Goudsmit and Uhlenbeck until 1925 (1925; 1926). One might say that electron spin was discovered before it was invented. (For details of this episode see Appendix 5 ).
In the last section we saw some of the difficulty inherent in experiment-theory comparison. One is sometimes faced with the question of whether the experimental apparatus satisfies the conditions required by theory, or conversely, whether the appropriate theory is being compared to the experimental result. A case in point is the history of experiments on the double-scattering of electrons by heavy nuclei (Mott scattering) during the 1930s and the relation of these results to Dirac’s theory of the electron, an episode in which the question of whether or not the experiment satisfied the conditions of the theoretical calculation was central. Initially, experiments disagreed with Mott’s calculation, casting doubt on the underlying Dirac theory. After more than a decade of work, both experimental and theoretical, it was realized that there was a background effect in the experiments that masked the predicted effect. When the background was eliminated experiment and theory agreed. ( Appendix 6 )
Ever vaster amounts of data have been produced by particle colliders as they have grown from room-size apparata, to tens of kilometers long mega-labs. Vast numbers of background interactions that are well understood and theoretically uninteresting occur in the detector. These have to be combed in order to identify interactions of potential interest. This is especially true of hadron (proton-proton) colliders like the Large Hadron Collider (LHC), where the Higgs boson was discovered. Protons that collide in the LHC and similar hadron colliders are composed of more elementary particles, collectively labeled partons. Partons mutually interact, exponentially increasing the number of background interactions. In fact, a minuscule number of interactions are selected from the overwhelming number that occur in the detector. (In contrast, lepton collisions, such as collisions of electrons and positrons, produce much lower backgrounds, since leptons are not composed of more elementary particles.)
Thus, a successful search for new elementary particles critically depends on successfully crafting selection criteria and techniques at the stage of data collection and at the stage of data analysis. But gradual development and changes in data selection procedures in the colliders raises an important epistemological concern. The main reason for this concern is nicely anticipated by the following question, which was posed by one of the most prominent experimentalists in particle physics: “What is the extent to which we are negating the discovery potential of very-high-energy proton machines by the necessity of rejecting, a priori, the events we cannot afford to record?” (Panofsky 1994, 133). In other words, how does one decide which interactions to detect and analyze in a multitude, in order to minimize the possibility of throwing out novel and unexplored ones?
One way of searching through vast amounts of data that are already in, i.e. those that the detector has already delivered, is to look for occurrences that remain robust under varying conditions of detection. Physicists employ the technique of data cuts in such analysis. They cut out data that may be unreliable—when, for instance, a data set may be an artefact rather than a genuine particle interaction the experimenters expect. E.g. a colliding beam may interact with the walls of the detector and not with the other colliding beam, while producing a signal identical to the signal the experimenters expected the beam-beam interaction to produce. Thus, if under various data cuts a result remains stable, then it is increasingly likely to be correct and to represent the genuine phenomenon the physicists think it represents. The robustness of the result under various data cuts minimizes the possibility that the detected phenomenon only mimics the genuine one (Franklin 2013, 224–5).
At the data-acquisition stage, however, this strategy does not seem applicable. As Panofsky suggests, one does not know with certainty which of the vast number of the events in the detector may be of interest.
Yet, Karaca (2011) [ 13 ] argues that a form of robustness is in play even at the acquisition stage. This experimental approach amalgamates theoretical expectations and empirical results, as the example of the hypothesis of specific heavy particles is supposed to illustrate.
Along with the Standard Model of particle physics, a number of alternative models have been proposed. Their predictions of how elementary particles should behave often differ substantially. Yet in contrast to the Standard Model, they all share the hypothesis that there exist heavy particles that decay into particles with high transverse momentum.
Physicists apply a robustness analysis in testing this hypothesis, the argument goes. First, they check whether the apparatus can detect known particles similar to those predicted. Second, guided by the hypothesis, they establish various trigger algorithms. (The trigger algorithms, or “the triggers”, determine at what exact point in time and under which conditions a detector should record interactions. They are necessary because the frequency and the number of interactions far exceed the limited recording capacity.) And, finally, they observe whether any results remain stable across the triggers.
Yet even in this theoretical-empirical form of robustness, as Franklin (2013, 225) points out, “there is an underlying assumption that any new physics will resemble known physics”—usually a theory of the day. And one way around this problem is for physicists to produce as many alternative models as possible, including those that may even seem implausible at the time.
Perovic (2011) suggests that such a potential failure, namely to spot potentially relevant events occurring in the detector, may be also a consequence of the gradual automation of the detection process.
The early days of experimentation in particle physics, around WWII, saw the direct involvement of the experimenters in the process. Experimental particle physics was a decentralized discipline where experimenters running individual labs had full control over the triggers and analysis. The experimenters could also control the goals and the design of experiments. Fixed target accelerators, where the beam hits the detector instead of another beam, produced a number of particle interactions that was manageable for such labs. The chance of missing an anomalous event not predicted by the current theory was not a major concern in such an environment.
Yet such labs could process a comparatively small amount of data. This has gradually become an obstacle, with the advent of hadron colliders. They work at ever-higher energies and produce an ever-vaster number of background interactions. That is why the experimental process has become increasingly automated and much more indirect. Trained technicians instead of experimenters themselves at some point started to scan the recordings. Eventually, these human scanners were replaced by computers, and a full automation of detection in hadron colliders has enabled the processing of vast number of interactions. This was the first significant change in the transition from small individual labs to mega-labs.
The second significant change concerned the organization and goals of the labs. The mega-detectors and the amounts of data they produced required exponentially more staff and scientists. This in turn led to even more centralized and hierarchical labs and even longer periods of design and performance of the experiments. As a result, focusing on confirming existing dominant hypotheses rather than on exploratory particle searches was the least risky way of achieving results that would justify unprecedented investments.
Now, an indirect detection process combined with mostly confirmatory goals is conducive to overlooking of unexpected interactions. As such, it may impede potentially crucial theoretical advances stemming from missed interactions.
This possibility that physicists such as Panofsky have acknowledged is not a mere speculation. In fact, the use of semi-automated, rather than fully-automated regimes of detection turned out to be essential for a number of surprising discoveries that led to theoretical breakthroughs.
Perovic (2011) analyzes several such cases, most notably the discovery of the J/psi particle that provided the first substantial piece of evidence for the existence of the charmed quark. In the experiments, physicists were able to perform exploratory detection and visual analysis of practically individual interactions due to low number of background interactions in the linear electron-positron collider. And they could afford to do this in an energy range that the existing theory did not recognize as significant, which led to them making the discovery. None of this could have been done in the fully automated detecting regime of hadron colliders that are indispensable when dealing with an environment that contains huge numbers of background interactions.
And in some cases, such as the Fermilab experiments that aimed to discover weak neutral currents, an automated and confirmatory regime of data analysis contributed to the failure to detect particles that were readily produced in the apparatus.
The complexity of the discovery process in particle physics does not end with concerns about what exact data should be chosen out of the sea of interactions. The so-called look-elsewhere effect results in a tantalizing dilemma at the stage of data analysis.
Suppose that our theory tells us that we will find a particle in an energy range. And suppose we find a significant signal in a section of that very range. Perhaps we should keep looking elsewhere within the range to make sure it is not another particle altogether we have discovered. It may be a particle that left other undetected traces in the range that our theory does not predict, along with the trace we found. The question is to what extent we should look elsewhere before we reach a satisfying level of certainty that it is the predicted particle we have discovered.
Physicists faced such a dilemma during the search for the Higgs boson at the Large Hadron Collider at CERN (Dawid 2015).
The Higgs boson is a particle responsible for the mass of other particles. It is a scalar field that “pulls back” moving and interacting particles. This pull, which we call mass, is different for different particles. It is predicted by the Standard Model, whereas alternative models predict somewhat similar Higgs-like particles.
A prediction based on the Standard Model tells us with high probability that we will find the Higgs particle in a particular range. Yet a simple and an inevitable fact of finding it in a particular section of that range may prompt us to doubt whether we have truly found the exact particle our theory predicted. Our initial excitement may vanish when we realize that we are much more likely to find a particle of any sort—not just the predicted particle—within the entire range than in a particular section of that range. Thus, the probability of finding the Higgs anywhere within a given energy range (consisting of eighty energy ‘bins’) is much higher than the probability of finding it at a particular energy scale within that range (i.e. in any individual bin). In fact, the likelihood of us finding it in a particular bin of the range is about hundred times lower.
In other words, the fact that we will inevitably find the particle in a particular bin, not only in a particular range, decreases the certainty that it was the Higgs we found. Given this fact alone we should keep looking elsewhere for other possible traces in the range once we find a significant signal in a bin. We should not proclaim the discovery of a particle predicted by the Standard Model (or any model for that matter) too soon. But for how long should we keep looking elsewhere? And what level of certainty do we need to achieve before we proclaim discovery?
The answer boils down to the weight one gives the theory and its predictions. This is the reason the experimentalists and theoreticians had divergent views on the criterion for determining the precise point at which they could justifiably state ‘Our data indicate that we have discovered the Higgs boson’. Theoreticians were confident that a finding within the range (any of eighty bins) that was of standard reliability (of three or four sigma), coupled with the theoretical expectations that Higgs would be found, would be sufficient. In contrast, experimentalists argued that at no point of data analysis should the pertinence of the look-elsewhere effect be reduced, and the search proclaimed successful, with the help of the theoretical expectations concerning Higgs. One needs to be as careful in combing the range as one practically may. As a result, the experimentalists’ preferred value of sigmas for announcing the discovery was five. This is a standard under which very few findings have turned out to be a fluctuation in the past.
Dawid argues that a question of an appropriate statistical analysis of data is at the heart of the dispute. The reasoning of the experimentalists relied on a frequentist approach that does not specify the probability of the tested hypothesis. It actually isolates statistical analysis of data from the prior probabilities. The theoreticians, however, relied on Bayesian analysis. It starts with prior probabilities of initial assumptions and ends with the assessment of the probability of tested hypothesis based on the collected evidence. The question remains whether the experimentalists’ reasoning was fully justified. The prior expectations that the theoreticians had included in their analysis had already been empirically corroborated by previous experiments after all.
3.4 Other Roles
Experiment can also provide us with evidence for the existence of the entities involved in our theories. J.J. Thomson’s experiments on cathode rays provided grounds for belief in the existence of electrons. (For details of this episode see Appendix 7 ).
Experiment can also help to articulate a theory. Experiments on beta decay during from the 1930s to the 1950s determined the precise mathematical form of Fermi’s theory of beta decay. (For details of this episode see Appendix 8 .)
The distinction between observation and experiment is relatively little discussed in philosophical literature, despite its continuous relevance to the scientific community and beyond in understanding specific traits and segments of the scientific process and the knowledge it produces.
Daston and her coauthors (Daston 2011; Daston and Lunbeck 2011; Daston and Galison 2007) have convincingly demonstrated that the distinction has played a role in delineating various features of scientific practice. It has helped scientists articulate their reflections on their own practice.
Observation is philosophically a loaded term, yet the epistemic status of scientific observation has evolved gradually with the advance of scientific techniques of inquiry and the scientific communities pursuing them. Daston succinctly summarizes this evolution in the following passage:
Characteristic of the emergent epistemic genre of the observations was, first, an emphasis on singular events, witnessed first hand (autopsia) by a named author (in contrast to the accumulation of anonymous data over centuries described by Cicero and Pliny as typical of observationes); second, a deliberate effort to separate observation from conjecture (in contrast to the medieval Scholastic connection of observation with the conjectural sciences, such as astrology); and third, the creation of virtual communities of observers dispersed over time and space, who communicated and pooled their observations in letters and publications (in contrast to passing them down from father to son or teacher to student as rare and precious treasures). (2011, 81)
Observation gradually became juxtaposed to other, more complex modes of inquiry such as experiment, “whose meaning shifted from the broad and heterogeneous sense of experimentum as recipe, trial, or just common experience to a concertedly artificial manipulation, often using special instruments and designed to probe hidden causes” (Daston 2011, 82).
In the 17th century, observation and experiment were seen as “an inseparable pair” (Daston 2011, 82) and by the 19th century they were understood to be essentially opposed, with the observer increasingly seen as passive and thus epistemically inferior to the experimenter. In fact, already Leibniz anticipated this view stating that “[t]here are certain experiments that would be better called observations, in which one considers rather than produces the work” (Daston 2011, 86). This aspect of the distinction has been a mainstay of understanding scientific practice ever since.
Shapere (1982) pointed out that the usage of the notion of observation is embedded in scientific practice, including testing and justification of scientific theories. Neutrinos arriving from the Sun are said to be observed in the detector but are also said to be an observation of the Sun’s core. Yet, the background knowledge provides the foundation for a meaningful distinction. One the one hand there are weakly interacting neutrinos leaving the Sun’s core and entering detector practically interrupted. This may be justifiably qualified as “direct observation” of the Sun’s core. On the other hand, there is a rather indirect observation of the core via detection of light-photons that travel for billions of years from the Sun’s core through the plasma to the electromagnetic detectors.
There are currently two prominent and opposed views of the experiment-observation distinction. Ian Hacking has characterized it as well-defined, while avoiding the claim that observation and experiment are opposites (Hacking 1983, 173). According to him, the notions signify different things in scientific practice. The experiment is a thorough manipulation that creates a new phenomenon, and observation of the phenomenon is its outcome. If scientists can manipulate a domain of nature to such an extent that they can create a new phenomenon in a lab, a phenomenon that normally cannot be observed in nature, then they have truly observed the phenomenon (Hacking 1989, 1992).
Meanwhile, other authors concur that “the familiar distinction between observation and experiment … [is] an artefact of the disembodied, reconstructed character of retrospective accounts” (Gooding 1992, 68). The distinction “collapses” when we are faced with actual scientific practice as a process, and “Hacking’s observation versus experiment framework does not survive intact when put to the test in a range of cases of scientific experimentation” (Malik 2017, 85). First, the uses of the distinction cannot be compared across scientific fields. And second, as Gooding (1992) suggests, observation is a process too, not simply a static result of manipulation. Thus, both observation and experiment are seen as concurrent processes blended together in scientific practice.
Malik (2017, 86) states that these arguments are the reason why “very few [authors] use Hacking’s nomenclature of observation/experiment” and goes so far to conclude that “to (try to) distinguish between observation and experiment is futile.” There is no point delineating the two except perhaps in certain narrow domains; e.g., Hacking’s notion of the experiment based on creating phenomena might be useful within a narrow domain of particle physics. (See also Chang 2011.) He advocates avoiding the distinction altogether and opting for “the terminology [that] underlines this sense of continuousness” (Malik 2017, 88) instead. If we want to analyze scientific practice, the argument goes, we should leave behind the idea of the distinction as fundamental and turn to the characterization and analysis of various “epistemic activities” instead, e.g., along the lines suggested by Chang (2011).
A rather obvious danger of this approach is an over-emphasis on the continuousness of the notions of observation and experiment that results in inadvertent equivocation. And this, in turn, results in sidelining the distinction and its subtleties in the analysis of the scientific practice, despite their crucial role in articulating and developing that practice since the 17th century. It is possible that these two notions form a continuum spreading along the axes of manipulability and accessibility of both target phenomena and observational conditions, and that the key points on such continuum define various evolving practices. Thus, each scientific practice may be located on the continuum, with the location defining both its epistemic properties and the epistemic (as well as ethical) obligations that underlie it at a given time. (Perović 2021)
On the one hand, the extent of the manipulation of phenomena and research conditions ranges from bodily manipulations (e.g. finding a convenient location to observe a planet with the naked eye) to the production of new phenomena in an elaborate apparatus like LHC at CERN, where the label “experiment” aims to delineate a substantial threshold of manipulation. On the other hand, depending on the background knowledge and aims of the research, observational accessibility ranges from mere detection of a potentially interesting phenomenon (e.g. a metal detector detects something in the ground), all the way to direct observation of the phenomenon’s properties (e.g., the microscopic analysis of a metal object).
5. Some Comparisons With Experiment in Biology
One comment that has been made concerning the philosophy of experiment is that all of the examples are taken from physics and are therefore limited. In this section arguments will be presented that these discussions also apply to biology.
Although all of the illustrations of the epistemology of experiment come from physics, David Rudge (1998; 2001) has shown that they are also used in biology. His example is Kettlewell’s (1955; 1956; 1958) evolutionary biology experiments on the Peppered Moth, Biston betularia . The typical form of the moth has a pale speckled appearance and there are two darker forms, f. carbonaria , which is nearly black, and f. insularia , which is intermediate in color. The typical form of the moth was most prevalent in the British Isles and Europe until the middle of the nineteenth century. At that time things began to change. Increasing industrial pollution had both darkened the surfaces of trees and rocks and had also killed the lichen cover of the forests downwind of pollution sources. Coincident with these changes, naturalists had found that rare, darker forms of several moth species, in particular the Peppered Moth, had become common in areas downwind of pollution sources.
Kettlewell attempted to test a selectionist explanation of this phenomenon. E.B. Ford (1937; 1940) had suggested a two-part explanation of this effect: 1) darker moths had a superior physiology and 2) the spread of the melanic gene was confined to industrial areas because the darker color made carbonaria more conspicuous to avian predators in rural areas and less conspicuous in polluted areas. Kettlewell believed that Ford had established the superior viability of darker moths and he wanted to test the hypothesis that the darker form of the moth was less conspicuous to predators in industrial areas.
Kettlewell’s investigations consisted of three parts. In the first part he used human observers to investigate whether his proposed scoring method would be accurate in assessing the relative conspicuousness of different types of moths against different backgrounds. The tests showed that moths on “correct” backgrounds, typical on lichen covered backgrounds and dark moths on soot-blackened backgrounds were almost always judged inconspicuous, whereas moths on “incorrect” backgrounds were judged conspicuous.
The second step involved releasing birds into a cage containing all three types of moth and both soot-blackened and lichen covered pieces of bark as resting places. After some difficulties (see Rudge 1998 for details), Kettlewell found that birds prey on moths in an order of conspicuousness similar to that gauged by human observers.
The third step was to investigate whether birds preferentially prey on conspicuous moths in the wild. Kettlewell used a mark-release-recapture experiment in both a polluted environment (Birmingham) and later in an unpolluted wood. He released 630 marked male moths of all three types in an area near Birmingham, which contained predators and natural boundaries. He then recaptured the moths using two different types of trap, each containing virgin females of all three types to guard against the possibility of pheromone differences.
Kettlewell found that carbonaria was twice as likely to survive in soot-darkened environments (27.5 percent) as was typical (12.7 percent). He worried, however, that his results might be an artifact of his experimental procedures. Perhaps the traps used were more attractive to one type of moth, that one form of moth was more likely to migrate, or that one type of moth just lived longer. He eliminated the first alternative by showing that the recapture rates were the same for both types of trap. The use of natural boundaries and traps placed beyond those boundaries eliminated the second, and previous experiments had shown no differences in longevity. Further experiments in polluted environments confirmed that carbonaria was twice as likely to survive as typical. An experiment in an unpolluted environment showed that typical was three times as likely to survive as carbonaria . Kettlewell concluded that such selection was the cause of the prevalence of carbonaria in polluted environments.
Rudge also demonstrates that the strategies used by Kettlewell are those described above in the epistemology of experiment. His examples are given in Table 1. (For more details see Rudge 1998).
1. | Experimental checks and calibration in which the apparatus reproduces known phenomena. | Use of the scoring experiment to verify that the proposed scoring methods would be feasible and objective. |
2. | Reproducing artifacts that are known in advance to be present. | Analysis of recapture figures for endemic populations. |
3. | Elimination of plausible sources of background and alternative explanations of the result. | Use of natural barriers to minimize migration. |
4. | Using the results themselves to argue for their validity. | Filming the birds preying on the moths. |
5. | Using an independently well-corroborated theory of the phenomenon to explain the results. | Use of Ford’s theory of the spread of industrial melanism. |
6. | Using an apparatus based on a well- corroborated theory. | Use of Fisher, Ford, and Shepard techniques. [The mark-release-capture method had been used in several earlier experiments] |
7. | Using statistical arguments. | Use and analysis of large numbers of moths. |
8. | Blind analysis | Not used. |
9. | Intervention, in which the experimenter manipulates the object under observation | Not present |
10. | Independent confirmation using different experiments. | Use of two different types of traps to recapture the moths. |
Table 1. Examples of epistemological strategies used by experimentalists in evolutionary biology, from H.B.D. Kettlewell’s (1955, 1956, 1958) investigations of industrial melanism. (See Rudge 1998).
The roles that experiment plays in physics are also those it plays in biology. In the previous section we have seen that Kettlewell’s experiments both test and confirm a theory. I discussed earlier a set of crucial experiments that decided between two competing classes of theories, those that conserved parity and those that did not. In this section I will discuss an experiment that decided among three competing mechanisms for the replication of DNA, the molecule now believed to be responsible for heredity. This is another crucial experiment. It strongly supported one proposed mechanism and argued against the other two. (For details of this episode see Holmes 2001.)
In 1953 Francis Crick and James Watson proposed a three-dimensional structure for deoxyribonucleic acid (DNA) (Watson and Crick 1953a). Their proposed structure consisted of two polynucleotide chains helically wound about a common axis. This was the famous “Double Helix”. The chains were bound together by combinations of four nitrogen bases — adenine, thymine, cytosine, and guanine. Because of structural requirements only the base pairs adenine-thymine and cytosine-guanine are allowed. Each chain is thus complementary to the other. If there is an adenine base at a location in one chain there is a thymine base at the same location on the other chain, and vice versa. The same applies to cytosine and guanine. The order of the bases along a chain is not, however, restricted in any way, and it is the precise sequence of bases that carries the genetic information.
The significance of the proposed structure was not lost on Watson and Crick when they made their suggestion. They remarked, “It has not escaped our notice that the specific pairing we have postulated immediately suggests a possible copying mechanism for the genetic material.”
If DNA was to play this crucial role in genetics, then there must be a mechanism for the replication of the molecule. Within a short period of time following the Watson-Crick suggestion, three different mechanisms for the replication of the DNA molecule were proposed (Delbruck and Stent 1957). These are illustrated in Figure A. The first, proposed by Gunther Stent and known as conservative replication, suggested that each of the two strands of the parent DNA molecule is replicated in new material. This yields a first generation which consists of the original parent DNA molecule and one newly-synthesized DNA molecule. The second generation will consist of the parental DNA and three new DNAs.

Figure A: Possible mechanisms for DNA replication. (Left) Conservative replication. Each of the two strands of the parent DNA is replicated to yield the unchanged parent DNA and one newly synthesized DNA. The second generation consists of one parent DNA and three new DNAs. (Center) Semiconservative replication. Each first generation DNA molecule contains one strand of the parent DNA and one newly synthesized strand. The second generation consists of two hybrid DNAs and two new DNAs. (Right) Dispersive replication. The parent chains break at intervals, and the parental segments combine with new segments to form the daughter chains. The darker segments are parental DNA and the lighter segments are newly synthesized DNA. From Lehninger (1975).
The second proposed mechanism, known as semiconservative replication is when each strand of the parental DNA acts as a template for a second newly-synthesized complementary strand, which then combines with the original strand to form a DNA molecule. This was proposed by Watson and Crick (1953b). The first generation consists of two hybrid molecules, each of which contains one strand of parental DNA and one newly synthesized strand. The second generation consists of two hybrid molecules and two totally new DNAs. The third mechanism, proposed by Max Delbruck, was dispersive replication, in which the parental DNA chains break at intervals and the parental segments combine with new segments to form the daughter strands.
In this section the experiment performed by Matthew Meselson and Franklin Stahl, which has been called “the most beautiful experiment in biology”, and which was designed to answer the question of the correct DNA replication mechanism will be discussed (Meselson and Stahl 1958). Meselson and Stahl described their proposed method. “We anticipated that a label which imparts to the DNA molecule an increased density might permit an analysis of this distribution by sedimentation techniques. To this end a method was developed for the detection of small density differences among macromolecules. By use of this method, we have observed the distribution of the heavy nitrogen isotope \(\ce{^{15}N}\) among molecules of DNA following the transfer of a uniformly \(\ce{^{15}N}\)-labeled, exponentially growing bacterial population to a growth medium containing the ordinary nitrogen isotope \(\ce{^{14}N}\)” (Meselson and Stahl 1958, pp. 671–672).

Figure B: Schematic representation of the Meselson-Stahl experiment. From Watson (1965).
The experiment is described schematically in Figure B. Meselson and Stahl placed a sample of DNA in a solution of cesium chloride. As the sample is rotated at high speed the denser material travels further away from the axis of rotation than does the less dense material. This results in a solution of cesium chloride that has increasing density as one goes further away from the axis of rotation. The DNA reaches equilibrium at the position where its density equals that of the solution. Meselson and Stahl grew E. coli bacteria in a medium that contained ammonium chloride \((\ce{NH4Cl})\) as the sole source of nitrogen. They did this for media that contained either \(\ce{^{14}N}\), ordinary nitrogen, or \(\ce{^{15}N}\), a heavier isotope. By destroying the cell membranes they could obtain samples of DNA which contained either \(\ce{^{14}N}\) or \(\ce{^{15}N}\). They first showed that they could indeed separate the two different mass molecules of DNA by centrifugation (Figure C). The separation of the two types of DNA is clear in both the photograph obtained by absorbing ultraviolet light and in the graph showing the intensity of the signal, obtained with a densitometer. In addition, the separation between the two peaks suggested that they would be able to distinguish an intermediate band composed of hybrid DNA from the heavy and light bands. These early results argued both that the experimental apparatus was working properly and that all of the results obtained were correct. It is difficult to imagine either an apparatus malfunction or a source of experimental background that could reproduce those results. This is similar, although certainly not identical, to Galileo’s observation of the moons of Jupiter or to Millikan’s measurement of the charge of the electron. In both of those episodes it was the results themselves that argued for their correctness.

Figure C: The separation of \(\ce{^{14}N}\) DNA from \(\ce{^{15}N}\) DNA by centrifugation. The band on the left is \(\ce{^{14}N}\) DNA and that on the right is from \(\ce{^{15}N}\) DNA. From Meselson and Stahl (1958).
Meselson and Stahl then produced a sample of E coli bacteria containing only \(\ce{^{15}N}\) by growing it in a medium containing only ammonium chloride with \(\ce{^{15}N}\) \((\ce{^{15}NH4Cl})\) for fourteen generations. They then abruptly changed the medium to \(\ce{^{14}N}\) by adding a tenfold excess of \(\ce{^{14}NH_4Cl}\). Samples were taken just before the addition of \(\ce{^{14}N}\) and at intervals afterward for several generations. The cell membranes were broken to release the DNA into the solution and the samples were centrifuged and ultraviolet absorption photographs taken. In addition, the photographs were scanned with a recording densitometer. The results are shown in Figure D, showing both the photographs and the densitometer traces. The figure shows that one starts only with heavy (fully-labeled) DNA. As time proceeds one sees more and more half-labeled DNA, until at one generation time only half-labeled DNA is present. “Subsequently only half labeled DNA and completely unlabeled DNA are found. When two generation times have elapsed after the addition of \(\ce{^{14}N}\) half-labeled and unlabeled DNA are present in equal amounts” (p. 676). (This is exactly what the semiconservative replication mechanism predicts). By four generations the sample consists almost entirely of unlabeled DNA. A test of the conclusion that the DNA in the intermediate density band was half labeled was provided by examination of a sample containing equal amounts of generations 0 and 1.9. If the semiconservative mechanism is correct then Generation 1.9 should have approximately equal amounts of unlabeled and half-labeled DNA, whereas Generation 0 contains only fully-labeled DNA. As one can see, there are three clear density bands and Meselson and Stahl found that the intermediate band was centered at \((50 \pm 2)\) percent of the difference between the \(\ce{^{14}N}\) and \(\ce{^{15}N}\) bands, shown in the bottom photograph (Generations 0 and 4.1). This is precisely what one would expect if that DNA were half labeled.

Figure D: (Left) Ultraviolet absorption photographs showing DNA bands from centrifugation of DNA from E. Coli sampled at various times after the addition of an excess of \(\ce{^{14}N}\) substrates to a growing \(\ce{^{15}N}\) culture. (Right) Densitometer traces of the photographs. The initial sample is all heavy (\(\ce{^{15}N}\) DNA). As time proceeds a second intermediate band begins to appear until at one generation all of the sample is of intermediate mass (Hybrid DNA). At longer times a band of light DNA appears, until at 4.1 generations the sample is almost all lighter DNA. This is exactly what is predicted by the Watson-Crick semiconservative mechanism. From Meselson and Stahl (1958)
Meselson and Stahl stated their results as follows, “The nitrogen of DNA is divided equally between two subunits which remain intact through many generations…. Following replication, each daughter molecule has received one parental subunit” (p. 676).
Meselson and Stahl also noted the implications of their work for deciding among the proposed mechanisms for DNA replication. In a section labeled “The Watson-Crick Model” they noted that, “This [the structure of the DNA molecule] suggested to Watson and Crick a definite and structurally plausible hypothesis for the duplication of the DNA molecule. According to this idea, the two chains separate, exposing the hydrogen-bonding sites of the bases. Then, in accord with base-pairing restrictions, each chain serves as a template for the synthesis of its complement. Accordingly, each daughter molecule contains one of the parental chains paired with a newly synthesized chain…. The results of the present experiment are in exact accord with the expectations of the Watson-Crick model for DNA replication” (pp. 677–678).
It also showed that the dispersive replication mechanism proposed by Delbruck, which had smaller subunits, was incorrect. “Since the apparent molecular weight of the subunits so obtained is found to be close to half that of the intact molecule, it may be further concluded that the subunits of the DNA molecule which are conserved at duplication are single, continuous structures. The scheme for DNA duplication proposed by Delbruck is thereby ruled out” (p. 681). Later work by John Cairns and others showed that the subunits of DNA were the entire single polynucleotide chains of the Watson-Crick model of DNA structure.
The Meselson-Stahl experiment is a crucial experiment in biology. It decided between three proposed mechanisms for the replication of DNA. It supported the Watson-Crick semiconservative mechanism and eliminated the conservative and dispersive mechanisms. It played a similar role in biology to that of the experiments that demonstrated the nonconservation of parity did in physics. Thus, we have seen evidence that experiment plays similar roles in both biology and physics and also that the same epistemological strategies are used in both disciplines.
One interesting recent development in science, and thus in the philosophy of science, has been the increasing use of, and importance of, computer simulations. In some fields, such as high-energy physics, simulations are an essential part of all experiments. It is fair to say that without computer simulations these experiments would be impossible. There has been a considerable literature in the philosophy of science discussing whether computer simulations are experiments, theory, or some new kind of hybrid method of doing science. But, as Eric Winsberg remarked, “We have in other words, rejected the overly conservative intuition that computer simulation is nothing but boring and straightforward theory application. But we have avoided embracing the opposite, overly grandiose intuition that simulation is a radically new kind of knowledge production, ”on a par“ with experimentation. In fact, we have seen that soberly locating simulation ”on the methodological map“ is not a simple matter (Winsberg 2010, p. 136).”
Given the importance of computer simulations in science it is essential that we have good reasons to believe their results. Eric Winsberg (2010), Wendy Parker (2008) and others have shown that scientists use strategies quite similar to those discussed in Section 1.1.1, to argue for the correctness of computer simulations.
In this entry varying views on the nature of experimental results have been presented. Some argue that the acceptance of experimental results is based on epistemological arguments, whereas others base acceptance on future utility, social interests, or agreement with existing community commitments. Everyone agrees , however, that for whatever reasons, a consensus is reached on experimental results. These results then play many important roles in physics and we have examined several of these roles, although certainly not all of them. We have seen experiment deciding between two competing theories, calling for a new theory, confirming a theory, refuting a theory, providing evidence that determined the mathematical form of a theory, and providing evidence for the existence of an elementary particle involved in an accepted theory. We have also seen that experiment has a life of its own, independent of theory. If, as I believe, epistemological procedures provide grounds for reasonable belief in experimental results, then experiment can legitimately play the roles I have discussed and can provide the basis for scientific knowledge.
- Ackermann, R., 1985. Data, Instruments and Theory , Princeton, N.J.: Princeton University Press.
- –––, 1991. “Allan Franklin, Right or Wrong”, PSA 1990 (Volume 2), A. Fine, M. Forbes and L. Wessels (ed.). East Lansing, MI: Philosophy of Science Association, 451–457.
- Adelberger, E.G., 1989. “High-Sensitivity Hillside Results from the Eot-Wash Experiment”, Tests of Fundamental Laws in Physics: Ninth Moriond Workshop , O. Fackler and J. Tran Thanh Van (ed.). Les Arcs, France: Editions Frontieres, 485–499.
- Anderson, M.H., J.R. Ensher, M.R. Matthews, et al ., 1995. “Observation of Bose-Einstein Condensation in a Dilute Atomic Vapor”. Science , 269: 198–201.
- Bell, J.S. and J. Perring, 1964. “2pi Decay of the K 2 o Meson”, Physical Review Letters , 13: 348–349.
- Bennett, W.R., 1989. “Modulated-Source Eotvos Experiment at Little Goose Lock”, Physical Review Letters , 62: 365–368.
- Bizzeti, P.G., A.M. Bizzeti-Sona, T. Fazzini, et al., 1989a. “Search for a Composition Dependent Fifth Force: Results of the Vallambrosa Experiment”, Tran Thanh Van, J. , O. Fackler (ed.). Gif Sur Yvette: Editions Frontieres.
- –––, 1989b. “Search for a Composition-dependent Fifth Force”, Physical Review Letters , 62: 2901–2904.
- Bogen, J. and Woodward J., 1988. “Saving the Phenomena”, The Philosophical Review , 97: 303–352.
- Bose, S., 1924. “Plancks Gesetz und Lichtquantenhypothese”. Zeitschrift für Physik , 26: 178–181.
- Burnett, K., 1995. “An Intimate Gathering of Bosons”. Science , 269: 182–183.
- Cartwright, N., 1983. How the Laws of Physics Lie , Oxford: Oxford University Press.
- Chang, H., 2004. Inventing temperature: Measurement and scientific progress . Oxford: Oxford University Press.
- Chang, H., 2007. "Scientific Progress: Beyond Foundationalism and Coherentism", Royal Institute of Philosophy Supplements , 61: 1–20.
- –––, 2011. “The philosophical grammar of scientific practice”, International Studies in the Philosophy of Science , 25: 205–221.
- Chase, C., 1929. “A Test for Polarization in a beam of Electrons by Scattering”, Physical Review , 34: 1069–1074.
- –––, 1930. “The Scattering of Fast Electrons by Metals. II. Polarization by Double Scattering at Right Angles”, Physical Review , 36: 1060–1065.
- Christenson, J.H., J.W. Cronin, V.L. Fitch, et al., 1964. “Evidence for the 2pi Decay of the \(\ce{K2^0}\) Meson”, Physical Review Letters , 13: 138–140.
- Collins, H., 1985. Changing Order: Replication and Induction in Scientific Practice , London: Sage Publications.
- –––, 1994. “A Strong Confirmation of the Experimenters’ Regress”, Studies in History and Philosophy of Modern Physics , 25(3): 493–503.
- Collins, H. and Pinch, T., 1993. The Golem: What Everyone Should Know About Science , Cambridge: Cambridge University Press.
- Conan Doyle, A., 1967. “The Sign of Four”, The Annotated Sherlock Holmes , W. S. Barrington-Gould (ed.). New York: Clarkson N. Potter.
- Cowsik, R., N. Krishnan, S.N. Tandor, et al., 1988. “Limit on the Strength of Intermediate-Range Forces Coupling to Isospin”. Physical Review Letters , 61: 2179–2181.
- –––, 1990. “Strength of Intermediate-Range Forces Coupling to Isospin”, Physical Review Letters , 64: 336–339.
- Daston, L., 2011. “The empire of observation”, in Histories of scientific observation , L. Daston and E. Lunbeck (eds.), Chicago: The University of Chicago Press, 81–113.
- Daston, L., and Galison, P., 2007. Objectivity , New York: Zone Books.
- Daston, L., and Lunbeck, E., 2011. Introduction, Histories of scientific observation , L. Daston and E. Lunbeck (eds.). Chicago: The University of Chicago Press, 1–9.
- Dawid, R., 2015. “Higgs Discovery and the Look-elsewhere Effect.” Philosophy of Science , 82(1): 76–96.
- de Groot, S.R. and H.A. Tolhoek, 1950. “On the Theory of Beta-Radioactivity I: The Use of Linear Combinations of Invariants in the Interaction Hamiltonian”, Physica , 16: 456–480.
- Delbruck, M. and G. S. Stent, 1957. On the Mechanism of DNA Replication. The Chemical Basis of Heredity. W. D. McElroy and B. Glass. Baltimore: Johns Hopkins Press: 699–736.
- Dymond, E.G., 1931. “Polarisation of a Beam of Electrons by Scattering”, Nature , 128: 149.
- –––, 1932. “On the Polarisation of Electrons by Scattering”, Proceedings of the Royal Society (London), A136: 638–651.
- –––, 1934. “On the Polarization of Electrons by Scattering. II.”, Proceedings of the Royal Society (London), A145: 657–668.
- Einstein, A., 1924. “Quantentheorie des einatomigen idealen Gases”, Sitzungsberischte der Preussische Akademie der Wissenschaften , Berlin, 261–267.
- –––, 1925. “Quantentheorie des einatomigen idealen gases”, Sitzungsberichte der Preussische Akadmie der Wissenschaften , Berlin, 3–14.
- Everett, A.E., 1965. “Evidence on the Existence of Shadow Pions in K + Decay”, Physical Review Letters , 14: 615–616.
- Fermi, E., 1934. “Attempt at a Theory of Beta-Rays”, Il Nuovo Cimento , 11: 1–21.
- Feynman, R.P. and M. Gell-Mann, 1958. “Theory of the Fermi Interaction”, Physical Review , 109: 193–198.
- Feynman, R.P., R.B. Leighton and M. Sands, 1963. The Feynman Lectures on Physics , Reading, MA: Addison-Wesley Publishing Company.
- Fierz, M., 1937. “Zur Fermischen Theorie des -Zerfalls”. Zeitschrift für Physik , 104: 553–565.
- Fischbach, E., S. Aronson, C. Talmadge, et al., 1986. “Reanalysis of the Eötvös Experiment”, Physical Review Letters , 56: 3–6.
- Fitch, V.L., 1981. “The Discovery of Charge-Conjugation Parity Asymmetry”, Science , 212: 989–993.
- Fitch, V.L., M.V. Isaila and M.A. Palmer, 1988. “Limits on the Existence of a Material-dependent Intermediate-Range Force”. Physical Review Letters , 60: 1801–1804.
- Ford, E. B., 1937. “Problems of Heredity in the Lepidoptera.” Biological Reviews , 12: 461–503.
- –––, 1940. “Genetic Research on the Lepidoptera.” Annals of Eugenics , 10: 227–252.
- Ford, K.W., 1968. Basic Physics , Lexington: Xerox.
- Franklin, A., 1986. The Neglect of Experiment , Cambridge: Cambridge University Press.
- –––, 1990. Experiment, Right or Wrong , Cambridge: Cambridge University Press.
- –––, 1993a. The Rise and Fall of the Fifth Force: Discovery, Pursuit, and Justification in Modern Physics , New York: American Institute of Physics.
- –––, 1993b. “Discovery, Pursuit, and Justification.” Perspectives on Science , 1: 252–284.
- –––, 1994. “How to Avoid the Experimenters’ Regress”, Studies in the History and Philosophy of Science 25: 97–121.
- –––, 1995. “Laws and Experiment”, Laws of Nature , F. Weinert (ed.). Berlin, De Gruyter:191–207.
- –––, 1997a. “Calibration”, Perspectives on Science , 5: 31–80.
- –––, 1997b. “Recycling Expertise and Instrumental Loyalty”, Philosophy of Science (Supplement), 64(4): S42–S52.
- –––, 2002. Selectivity and Discord: Two Problems of Experiment , Pittsburgh: University of Pittsburgh Press.
- –––, 2013. Shifting Standards: Experiments in Particle Physics in the Twentieth Century , Pittsburgh: University of Pittsburgh Press.
- Franklin, A. and C. Howson, 1984. “Why Do Scientists Prefer to Vary Their Experiments?”, Studies in History and Philosophy of Science , 15: 51–62.
- Franklin, A., et al., 1989. “Can a theory-laden observation test the theory?”, British Journal for the Philosophy of Science , 40(2): 229–231.
- Friedman, J.L. and V.L. Telegdi, 1957. “Nuclear Emulsion Evidence for Parity Nonconservation in the Decay Chain \(pi^+ -- \mu^+ -- e^+\)”, Physical Review , 105(5): 1681–1682.
- Galison, P., 1987. How Experiments End , Chicago: University of Chicago Press.
- –––, 1997. Image and Logic , Chicago: University of Chicago Press.
- Gamow, G. and E. Teller, 1936. “Selection Rules for the -Disintegration”, Physical Review , 49: 895–899.
- Garwin, R.L., L.M. Lederman and M. Weinrich, 1957. “Observation of the Failure of Conservation of Parity and Charge Conjugation in Meson Decays: The Magnetic Moment of the Free Muon”, Physical Review 105: 1415–1417.
- Gerlach, W. and O. Stern, 1922a. “Der experimentelle Nachweis der Richtungsquantelung”, Zeitschrift fur Physik , 9: 349–352.
- –––, 1924. “Uber die Richtungsquantelung im Magnetfeld”, Annalen der Physik , 74: 673–699.
- Glashow, S., 1992. “The Death of Science?” The End of Science? Attack and Defense , R.J. Elvee. Lanham, MD.: University Press of America
- Gooding, D., 1992. “Putting Agency Back Into Experiment”, in Science as Practice and Culture , A. Pickering (ed.). Chicago, University of Chicago Press, 65–112.
- Hacking, I., 1981. “Do We See Through a Microscope”, Pacific Philosophical Quarterly , 63: 305–322.
- –––, 1983. Representing and Intervening , Cambridge: Cambridge University Press.
- –––, 1989. “Extragalactic reality: The case of gravitational lensing”, Philosophy of Science , 56: 555–581.
- –––, 1992. “The Self-Vindication of the Laboratory Sciences”, Science as Practice and Culture , A. Pickering (ed.). Chicago, University of Chicago Press:29–64.
- –––, 1999. The Social Construction of What? Cambridge, MA: Harvard University Press.
- Halpern, O. and J. Schwinger, 1935. “On the Polarization of Electrons by Double Scattering”, Physical Review , 48: 109–110.
- Hamilton, D.R., 1947. “Electron-Neutrino Angular Correlation in Beta-Decay”, Physical Review , 71: 456–457.
- Hellmann, H., 1935. “Bemerkung zur Polarisierung von Elektronenwellen durch Streuung”, Zeitschrift fur Physik , 96: 247–250.
- Hermannsfeldt, W.B., R.L. Burman, P. Stahelin, et al., 1958. “Determination of the Gamow-Teller Beta-Decay Interaction from the Decay of Helium-6”, Physical Review Letters , 1: 61–63.
- Holmes, F. L., 2001. Meselson, Stahl, and the Replication of DNA, A History of “The Most Beautiful Experiment in Biology” , New Haven: Yale University Press.
- Karaca, K., 2011. “Progress Report 2 – Project: The Epistemology of the LHC.” Franklin A. Wuppertal, DE.
- –––, 2013. “The strong and weak senses of theory-ladenness of experimentation: Theory-driven versus exploratory experiments in the history of high-energy particle physics”. Science in Context , 26(1): 93–136.
- Kettlewell, H. B. D., 1955. “Selection Experiments on Industrial Melanism in the Lepidoptera.” Heredity , 9: 323–342.
- –––, 1956. “Further Selection Experiments on Industrial Melanism in the Lepidoptera.” Heredity 10 : 287–301.
- –––, 1958. “A Survey of the Frequencies of Biston betularia (L.) (Lep.) and its Melanic Forms in Great Britain.” Heredity , 12: 51–72.
- Kofoed-Hansen, O., 1955. “Neutrino Recoil Experiments”, Beta- and Gamma-Ray Spectroscopy , K. Siegbahn (ed.). New York, Interscience:357–372.
- Konopinski, E. and G. Uhlenbeck, 1935. “On the Fermi Theory of Radioactivity”, Physical Review , 48: 7–12.
- Konopinski, E.J. and L.M. Langer, 1953. “The Experimental Clarification of the Theory of –Decay”, Annual Reviews of Nuclear Science , 2: 261–304.
- Konopinski, E.J. and G.E. Uhlenbeck, 1941. “On the Theory of Beta-Radioactivity”, Physical Review , 60: 308–320.
- Langer, L.M., J.W. Motz and H.C. Price, 1950. “Low Energy Beta-Ray Spectra: Pm 147 S 35 ”, Physical Review , 77: 798–805.
- Langer, L.M. and H.C. Price, 1949. “Shape of the Beta-Spectrum of the Forbidden Transition of Yttrium 91”, Physical Review , 75: 1109.
- Langstroth, G.O., 1932. “Electron Polarisation”, Proceedings of the Royal Society (London), A136: 558–568.
- LaRue, G.S., J.D. Phillips, and W.M. Fairbank, DATE. “Observation of Fractional Charge of (1/3) e on Matter”, Physical Review Letters , 46: 967–970.
- Latour, B. and S. Woolgar, 1979. Laboratory Life: The Social Construction of Scientific Facts , Beverly Hills: Sage.
- –––, 1986. Laboratory Life: The Construction of Scientific Facts , Princeton: Princeton University Press.
- Lee, T.D. and C.N. Yang, 1956. “Question of Parity Nonconservation in Weak Interactions”, Physical Review , 104: 254–258.
- Lehninger, A. L., 1975. Biochemistry , New York: Worth Publishers.
- Lynch, M., 1991. “Allan Franklin’s Transcendental Physics.” PSA 1990, Volume 2 , A. Fine, M. Forbes, and L. Wessels. East Lansing, MI: Philosophy of Science Association, 2: 471–485.
- MacKenzie, D., 1989. “From Kwajelein to Armageddon? Testing and the Social Construction of Missile Accuracy”, The Uses of Experiment , D. Gooding, T. Pinch and S. Shaffer (ed.). Cambridge, Cambridge University Press, 409–435.
- Malik, S., 2017. “ Observation Versus Experiment: An Adequate Framework for Analysing Scientific Experimentation? ”. Journal for General Philosophy of Science , 48: 71–95.
- Mayer, M.G., S.A. Moszkowski and L.W. Nordheim, 1951. “Nuclear Shell Structure and Beta Decay. I. Odd A Nuclei”, Reviews of Modern Physics , 23: 315–321.
- McKinney, W., 1992. Plausibility and Experiment: Investigations in the Context of Pursuit. History and Philosophy of Science. Bloomington, IN, Indiana.
- Mehra, J. and H. Rechenberg, 1982. The Historical Development of Quantum Theory , New York: Springer-Verlag.
- Meselson, M. and F. W. Stahl, 1958. “The Replication of DNA in Escherichia Coli.” Proceedings of the National Academy of Sciences (U.S.A.), 44: 671–682.
- Millikan, R.A., 1911. “The Isolation of an Ion, A Precision Measurement of Its Charge, and the Correction of Stokes’s Law”. Physical Review , 32: 349–397.
- Morrison, M., 1990. “Theory, Intervention, and Realism”. Synthese , 82: 1–22.
- Mott, N.F., 1929. “Scattering of Fast Electrons by Atomic Nuclei”, Proceedings of the Royal Society (London), A124: 425–442.
- –––, 1931. “Polarization of a Beam of Electrons by Scattering”, Nature , 128(3228): 454.
- Nelson, A., 1994. “How Could Scientific Facts be Socially Constructed?”, Studies in History and Philosophy of Science , 25(4): 535–547.
- –––, 1932. “Tha Polarisation of Electrons by Double Scattering”, Proceedings of the Royal Society (London), A135: 429–458.
- Nelson, P.G., D.M. Graham and R.D. Newman, 1990. “Search for an Intermediate-Range Composition-dependent Force Coupling to N-Z”. Physical Review D , 42: 963–976.
- Newman, R., D. Graham and P. Nelson, 1989. “A ”Fifth Force“ Search for Differential Acceleration of Lead and Copper toward Lead”, in Tests of Fundamental Laws in Physics: Ninth Moriond Workshop , O. Fackler and J. Tran Thanh Van (ed.), Les Arcs: Editions Frontieres, 459–472.
- Nishijima, K. and M.J. Saffouri, 1965. “CP Invariance and the Shadow Universe”, Physical Review Letters , 14: 205–207.
- Pais, A., 1982. Subtle is the Lord… , Oxford: Oxford University Press.
- Panofsky, W., 1994. Particles and Policy (Masters of Modern Physics), New York: American Institute of Physics.
- Parker, W., 2008. “Franklin, Holmes, and the Epistmology of Computer Simulation.” International Studies in the Philosophy of Science , 22: 165–183.
- Pauli, W., 1933. “Die Allgemeinen Prinzipien der Wellenmechanik”, Handbuch der Physik , 24: 83–272.
- Perovic, S., 2006. “Schrödinger’s interpretation of quantum mechanics and the relevance of Bohr’s experimental critique”, Studies in History and Philosophy of Science Part B (Studies in History and Philosophy of Modern Physics), 37 (2): 275–297.
- –––, 2011. “Missing experimental challenges to the Standard Model of particle physics”, Studies in History and Philosophy of Science Part B (Studies in History and Philosophy of Modern Physics), 42 (1): 32–42.
- –––, 2013. “Emergence of complementarity and the Baconian roots of Niels Bohr’s method”, Studies in History and Philosophy of Science Part B (Studies in History and Philosophy of Modern Physics), 44 (3): 162–173.
- –––, 2017. “Experimenter’s regress argument, empiricism, and the calibration of the large hadron collider”, Synthese , 194: 313–332.
- Petschek, A.G. and R.E. Marshak, 1952. “The \(\beta\)-Decay of Radium E and the Pseusoscalar Interaction”, Physical Review , 85(4): 698–699.
- Pickering, A., 1981. “The Hunting of the Quark”, Isis , 72: 216–236.
- –––, 1984a. Constructing Quarks , Chicago: University of Chicago Press.
- –––, 1984b. “Against Putting the Phenomena First: The Discovery of the Weak Neutral Current”, Studies in the History and Philosophy of Science , 15: 85–117.
- –––, 1987. “Against Correspondence: A Constructivist View of Experiment and the Real”, PSA 1986 , A. Fine and P. Machamer (ed.). Pittsburgh, Philosophy of Science Association. 2: 196–206.
- –––, 1989. “Living in the Material World: On Realism and Experimental Practice”, in The Uses of Experiment , D. Gooding, T. Pinch and S. Schaffer (eds.), Cambridge, Cambridge University Press, 275–297.
- –––, 1991. “Reason Enough? More on Parity Violation Experiments and Electroweak Gauge Theory”, in PSA 1990 (Volume 2), A. Fine, M. Forbes, and L. Wessels (eds.), East Lansing, MI: Philosophy of Science Association, 2: 459–469.
- –––, 1995. The Mangle of Practice , Chicago: University of Chicago Press.
- Prentki, J., 1965. CP Violation , in Proceedings: Oxford International Conference on Elementary Particles (Oxford, UK, Sept 19–25, 1965), R.G. Moorhouse, A.E. Taylor, and T.R. Walsh (eds.), 47–58.
- Pursey, D.L., 1951. “The Interaction in the Theory of Beta Decay”, Philosophical Magazine , 42: 1193–1208.
- Raab, F.J., 1987. “Search for an Intermediate-Range Interaction: Results of the Eot-Wash I Experiment”, New and Exotic Phenomena: Seventh Moriond Workshop , O. Fackler and J. Tran Thanh Van (eds.), Les Arcs: Editions Frontieres: 567–577.
- Randall, H.M., R.G. Fowler, N. Fuson, et al., 1949. Infrared Determination of Organic Structures , New York: Van Nostrand.
- Richter, H., 1937. “Zweimalige Streuung schneller Elektronen”. Annalen der Physik , 28: 533–554.
- Ridley, B.W., 1954. Nuclear Recoil in Beta Decay. Physics , Ph. D. Dissertation, Cambridge University.
- Rose, M.E. and H.A. Bethe, 1939. “On the Absence of Polarization in Electron Scattering”, Physical Review , 55: 277–289.
- Rudge, D. W., 1998. “A Bayesian Analysis of Strategies in Evolutionary Biology.” Perspectives on Science , 6: 341–360.
- –––, 2001. “Kettlewell from an Error Statistician’s Point of View”, Perspectives on Science , 9: 59–77.
- Rupp, E., 1929. “Versuche zur Frage nach einer Polarisation der Elektronenwelle”, Zeitschrift fur Physik , 53: 548–552.
- –––, 1930a. “Ueber eine unsymmetrische Winkelverteilung zweifach reflektierter Elektronen”, Zeitschrift fur Physik , 61: 158–169.
- –––, 1930b. “Ueber eine unsymmetrische Winkelverteilung zweifach reflektierter Elektronen”, Naturwissenschaften , 18: 207.
- –––, 1931. “Direkte Photographie der Ionisierung in Isolierstoffen”, Naturwissenschaften , 19: 109.
- –––, 1932a. “Versuche zum Nachweis einer Polarisation der Elektronen”, Physickalsche Zeitschrift , 33: 158–164.
- –––, 1932b. “Neure Versuche zur Polarisation der Elektronen”, Physikalische Zeitschrift , 33: 937–940.
- –––, 1932c. “Ueber die Polarisation der Elektronen bei zweimaliger 90 o –Streuung”, Zeitschrift fur Physik , 79: 642–654.
- –––, 1934. “Polarisation der Elektronen an freien Atomen”, Zeitschrift fur Physik , 88: 242–246.
- Rustad, B.M. and S.L. Ruby, 1953. “Correlation between Electron and Recoil Nucleus in He 6 Decay”, Physical Review , 89: 880–881.
- –––, 1955. “Gamow-Teller Interaction in the Decay of He 6 ”, Physical Review , 97: 991–1002.
- Sargent, B.W., 1932. “Energy Distribution Curves of the Disintegration Electrons”, Proceedings of the Cambridge Philosophical Society , 24: 538–553.
- –––, 1933. “The Maximum Energy of the -Rays from Uranium X and other Bodies”, Proceedings of the Royal Society (London), A139: 659–673.
- Sauter, F., 1933. “Ueber den Mottschen Polarisationseffekt bei der Streuun von Elektronen an Atomen”, Annalen der Physik , 18: 61–80.
- Shapin, S. and Simon S., 1989. Leviathan and the Air-Pump: Hobbes, Boyle, and the Experimental Life , Princeton: Princeton University Press.
- Schindler, S., 2011. “Bogen and Woodward’s data-phenomena distinction, forms of theory-ladenness, and the reliability of data ”, Synthese , 182(1): 39–55.
- Sellars, W., 1962. Science, Perception, and Reality , New York: Humanities Press.
- Sherr, R. and J. Gerhart, 1952. “Gamma Radiation of C 10 ”. Physical Review , 86: 619.
- Sherr, R., H.R. Muether and M.G. White, 1949. “Radioactivity of C 10 and O 14 ”, Physical Review , 75: 282–292.
- Smith, A.M., 1951. “Forbidden Beta-Ray Spectra”, Physical Review , 82: 955–956.
- Staley, K., 1999 “Golden Events and Statistics: What’s Wrong with Galison’s Image/Logic Distinction.” Perspectives on Science , 7: 196–230.
- Stern, O., 1921. “Ein Weg zur experimentellen Prufung Richtungsquantelung im Magnet feld”, Zeitschrift fur Physik , 7: 249–253.
- Stubbs, C.W., E.G. Adelberger, B.R. Heckel, et al., 1989. “Limits on Composition-dependent Interactions using a Laboratory Source: Is There a ”Fifth Force?“”, Physical Review Letters , 62: 609–612.
- Stubbs, C.W., E.G. Adelberger, F.J. Raab, et al., 1987. “Search for an Intermediate-Range Interaction”, Physical Review Letters , 58: 1070–1073.
- Sudarshan, E.C.G. and R.E. Marshak, 1958. “Chirality Invariance and the Universal Fermi Interaction”, Physical Review , 109: 1860–1862.
- Tal, E., 2016. “Making time: A study in the epistemology of measurement”, The British Journal for the Philosophy of Science , 61(1): 297–335.
- –––, 2017a. “A model-based epistemology of measurement”, in Nicola Mößner and Alfred Nordmann (eds.), Reasoning in measurement , Routledge: 245–265.
- –––, 2017b. “Calibration: Modelling the measurement process”, Studies in History and Philosophy of Science Part A , 65: 33–45.
- Thieberger, P., 1987a. “Search for a Substance-Dependent Force with a New Differential Accelerometer”, Physical Review Letters , 58: 1066–1069.
- Thomson, G.P., 1933. “Polarisation of Electrons”, Nature , 132: 1006.
- –––, 1934. “Experiment on the Polarization of Electrons”, Philosophical Magazine , 17: 1058–1071.
- Thomson, J.J., 1897. “Cathode Rays”, Philosophical Magazine , 44: 293–316.
- Uhlenbeck, G.E. and S. Goudsmit, 1925. “Ersetzung der Hypothese von unmechanischen Zwang durch eine Forderung bezuglich des inneren Verhaltens jedes einzelnen Elektrons”, Naturwissenschaften , 13: 953–954.
- –––, 1926. “Spinning Electrons and the Structure of Spectra”, Nature , 117: 264–265.
- van Fraassen, B., 1980. The Scientific Image , Oxford: Clarendon Press.
- Watson, J. D., 1965. Molecular Biology of the Gene , New York: W.A. Benjamin, Inc.
- Watson, J. D. and F. H. C. Crick, 1953a. “A Structure for Deoxyribose Nucleic Acid”, Nature , 171: 737.
- –––, 1953b. “Genetical Implications of the Structure of Deoxyribonucleic Acid”, Nature , 171: 964–967.
- Weinert, F., 1995. “Wrong Theory—Right Experiment: The Significance of the Stern-Gerlach Experiments”, Studies in History and Philosophy of Modern Physics , 26B(1): 75–86.
- Winter, J., 1936. “Sur la polarisation des ondes de Dirac”, Academie des Science, Paris, Comptes rendus hebdomadaires des seances , 202: 1265–1266.
- Winsberg, E., 2010. Science in the Age of Computer Simulation , Chicago: University of Chicago Press.
- Wu, C.S., 1955. “The Interaction in Beta-Decay”, in Beta- and Gamma-Ray Spectroscopy , K. Siegbahn (ed.), New York, Interscience: 314–356.
- Wu, C.S., E. Ambler, R.W. Hayward, et al., 1957. “Experimental Test of Parity Nonconservation in Beta Decay”, Physical Review , 105: 1413–1415.
- Wu, C.S. and A. Schwarzschild, 1958. A Critical Examination of the He 6 Recoil Experiment of Rustad and Ruby. New York, Columbia University.
- Ackermann, R., 1988. “Experiments as the Motor of Scientific Progress”, Social Epistemology , 2: 327–335.
- Batens, D. and J.P. Van Bendegem (eds.), 1988. Theory and Experiment , Dordrecht: D. Reidel Publishing Company.
- Burian, R. M., 1992. “How the Choice of Experimental Organism Matters: Biological Practices and Discipline Boundaries”, Synthese , 92: 151–166.
- –––, 1993. “How the Choice of Experimental Organism Matters: Epistemological Reflections on an Aspect of Biological Practice”, Journal of the History of Biology , 26: 351–367.
- –––, 1993b. “Technique, Task Definition, and the Transition from Genetics to Molecular Genetics: Aspects of the Work on Protein Synthesis in the Laboratories of J. Monod and P. Zamecnik”, Journal of the History of Biology , 26: 387–407.
- –––, 1995. “Comments on Rheinberger”, in Concepts, Theories, and Rationality in the Biological Sciences , G. Wolters, J. G. Lennox and P. McLaughlin (eds.), Pittsburgh: University of Pittsburgh Press: 123–136.
- Franklin, A., 2018. Is It the Same Result? Replication in Physics . San Rafael, CA: Morgan and Claypool.
- Franklin, A. and R. Laymon, 2019. Measuring Nothing, Repeatedly , San Rafael, CA: Morgan and Claypool.
- –––, 2021. Once Can Be Enough: Decisive Experiments. No Replication Required , Heidelberg: Springer.
- Gooding, D., 1990. Experiment and the Making of Meaning , Dordrecht: Kluwer Academic Publishers.
- Gooding, D., T. Pinch and S. Schaffer (eds.), 1989. The Uses of Experiment , Cambridge: Cambridge University Press.
- Koertge, N. (ed.), 1998. A House Built on Sand: Exposing Postmodernist Myths About Science , Oxford: Oxford University Press.
- Pickering, A. (ed.), 1992. Science as Practice and Culture , Chicago: University of Chicago Press.
- Pinch, T., 1986. Confronting Nature , Dordrecht: Reidel.
- Rasmussen, N., 1993. “Facts, Artifacts, and Mesosomes: Practicing Epistemology with the Electron Microscope”, Studies in History and Philosophy of Science , 24: 227–265.
- Rheinberger, H.-J., 1997. Toward a History of Epistemic Things , Stanford: Stanford University Press.
- Shapere, D., 1982. “The Concept of Observation in Science and Philosophy”, Philosophy of Science , 49: 482–525.
- Weber, M., 2005. Philosophy of Experimental Biology , Cambridge: Cambridge University Press.
How to cite this entry . Preview the PDF version of this entry at the Friends of the SEP Society . Look up topics and thinkers related to this entry at the Internet Philosophy Ontology Project (InPhO). Enhanced bibliography for this entry at PhilPapers , with links to its database.
[Please contact the authors with suggestions.]
confirmation | logic: inductive | rationalism vs. empiricism | scientific method | scientific realism
Acknowledgments
We are grateful to Professor Carl Craver for both his comments on the manuscript and for his suggestions for further reading.
Copyright © 2023 by Allan Franklin < allan . franklin @ colorado . edu > Slobodan Perovic < sperovic @ f . bg . ac . rs >
- Accessibility
Support SEP
Mirror sites.
View this site from another server:
- Info about mirror sites
The Stanford Encyclopedia of Philosophy is copyright © 2023 by The Metaphysics Research Lab , Department of Philosophy, Stanford University
Library of Congress Catalog Data: ISSN 1095-5054
- Skip to main navigation
- Skip to search
- Skip to content
- Press Release Archive
- AAPT Committees
- Area Committee Nomination Form
- Area Committees
- Annual Report
- Job Opportunities
- Code of Conduct
- Mission Statement
Organization
- Marketing Opportunities
- Privacy Statement
- Strategic Plan
- AAPT's DEI Strategy
- COMMUNITIES
- 2025 AAPT Winter Meeting
- 2024 AAPT Summer Meeting
- National Meetings
- Highlights of Past Meetings
- Meeting Abstract Archive
- TYC Tandem Meeting
- Physics Department Chairs Conference
- Awards & Medals
- Collaborative Projects
- U.S. Physics Team
- Grants & Scholarships
- Contests & Competitions
- New Faculty Programs
- K-12 Portal
- Virtual Coffee Hour
- Publications Information
- AJP Website
- AAPT Book Archive
- Browse AJP Online
- Advertising Media Kit
- Browse TPT Online
Video Abstracts
- The Physics Teacher TOC
- TOC Archive
- eNNOUNCER Archive
- AAPT Annual Report
- Physical Review Physics Education Research
- Physics Today
- AAPT Section News Archive
- News Archive
- AAPT ComPADRE Digital Library
- Joining AAPT - Levels & Dues
- Member Benefits
- Renew your membership
- View or update your profile
- Member Directory
- How to Get Involved with AAPT
- Testimonials
- Member Spotlight Archive
- In Memoriam
- Diversity, Equity, and Inclusion in Physics
- Article Collections
- Sustainability in Physics
- Colleges and Universities
- AAPT ComPADRE Digital library
- Speakers Bureau
- AAPT Career Center
- Program Review
- Media Relations
- Policy & Legislation
- Guidelines and Recommendations
- AAPT Sections
- Affiliated Organizations
- PERTG & PERLOC
- Supporters of AAPT
- Planned Giving
- Volunteering
- Publications & Journals ›
- Contributors ›
- - Formatting the Manuscript
AJP Section Navigation Show navigation
- Journal Description
- Editorial Staff
- Statement of Editorial Policy
- Current Table of Contents
- Information
- Submissions Procedures
- Formatting the Manuscript
- Formatting Figures
- General Information
- Review Procedures
- Anonymous Review
- Collections
- Resource Letters
- Computational Physics
- Back of the Envelope
- Theme Issues/Memorable Papers
- Journal Editorial History
American Journal of Physics ®
A publication of the american association of physics teachers ®.
- About the journal
- Contributor
General Info | "); document.writeln(" Submissions |"); document.writeln(" Manuscript Format | "); document.writeln(" Figure Preparation | "); document.writeln(" Editorial Procedures
This page describes how to format a manuscript for submission to AJP. Be sure to also read the submission requirements and procedures .
Electronic File Formats
- For initial submissions we require a single .pdf file containing all text, endnotes, figures, and tables. You may use any software you like to prepare this file, but to avoid extensive reworking later on, we strongly suggest that you use LaTeX/REVTeX or Microsoft Word, as described below. The ability to create a .pdf file is built into the Macintosh operating system and is also available in most LaTeX processors and in recent versions of MSWord. For advice on creating a .pdf in older software environments, see https://editorialexpress.com/e-editor/pdftips.html . If at all possible, your .pdf file should be no larger than one megabyte in size.
- The preferred editable format is LaTeX, using the REVTeX 4.1 style. For typical article submissions with many equations and/or endnotes, LaTeX/REVTeX is strongly recommended because it handles most of the formatting and numbering automatically. It also handles equations extremely well, making them easy to enter and producing beautiful typeset output. LaTeX is free software, available for all major operating systems. See the LaTeX web site for links and downloading instructions. We recommend that you get a “complete TeX installation,” which will include the REVTeX 4.1 style and all needed fonts, packages, and GUI tools. Alternatively, you may wish to try a cloud-based LaTeX processor such as writeLaTeX or ShareLaTeX . For a tutorial on using LaTeX, see our sample manuscript file .
- The only acceptable alternative to LaTeX is Microsoft Word .docx format ( not .doc). Authors using MSWord will have to pay especially careful attention to the detailed formatting instructions below. Do not use the “track changes” feature of MSWord. Be sure to use only the built-in MSWord equation editor (do not, e.g., use MathType), and use only standard fonts. We do not recommend MSWord for papers that contain many equations or long reference lists. However, for manuscripts that are especially short and simple, MSWord may be more convenient than LaTeX.
- The appropriate format for figures depends on their content and on whether they are part of an initial submission or an editable package for production. See our detailed instructions for figure preparation .
Sample Manuscript File
Authors are urged to use LaTeX to prepare their manuscripts, although MSWord is an acceptable alternative. The sample manuscript pdf and sample manuscript files are designed to provide a useful tutorial and template for LaTeX submissions for both new users and experts. The sample manuscript files are a .zip archive containing the LaTeX source file and two figure files that are required to produce the finished .pdf file.
General Style
The Style Manual of the American Institute of Physics, 4th ed. (1990) contains a wealth of information on the preparation of manuscripts, including advice on good writing and organization; rules for punctuation, capitalization, English usage, and using mathematical expressions; and lists of standard spellings and abbreviations. While advancing technology has made some parts of this document out of date, and a few of its rules are superseded by AJP's special style conventions, the Style Manual is still the authoritative reference unless there is a conflict with information appearing on the AJP website, in which case the latter takes precedence. Like other journals published in the U.S., AJP uses American rather than British spellings : color rather than colour; analyze rather than analyse; and so on. Authors are encouraged to use SI units, but use of SI units is not mandatory if other units are more appropriate. Authors are expected to word their manuscripts in a manner consistent with the fact that the physicists, students, and teachers who read AJP include genders other than male; the use of "they" as a singular pronoun is acceptable.
Manuscripts can be single- or double-spaced and single- or double-column. Reviewers appreciate text that is formatted for easy reading. All manuscript pages should be numbered. You will be asked to upload a single pdf file containing all text, endnotes, figures, and tables, preferably with the figures and tables incorporated into the text rather than at the end. Ensure that your manuscript meets the requirements for anonymous review .
Authors should make every effort to be concise. Generally speaking, readership and length are inversely related. Longer manuscripts will be subject to higher expectations with respect to the interest and usefulness of their content during the review process. Additional examples, further implications, and longer derivations can be placed in the easily-accessible online supplementary material.
AJP papers should normally be 4000 to 6000 words, plus equations, tables, and figures if appropriate. As a rough rule, a double-spaced, 12-point manuscript of length x pages (including figures and equations) will require x/3 journal pages to print, and we aim for papers to be 6 pages or shorter. Longer papers can be published, but their additional length should be justified by their high interest to readers. Note that dividing a paper into two linked papers does not solve the problem of a too-long paper, since the length restrictions are aimed at limiting the total number of pages that are dedicated to a particular topic, in order to allow more breadth of coverage in the journal.
Manuscripts intended for the Notes and Discussions section should be considerably shorter, typically 1000 to 3000 words, and Letters should normally be 800 words or shorter.
The main elements of an AJP paper are: Title Authors and affiliations (these are omitted to allow anonymous review until the manuscript is conditionally accepted) Abstract (optional for the Notes and Discussions section) Introductory section Main body, divided into sections and subsections as appropriate Concluding section (optional – don't simply write a summary) Acknowledgments (optional, and omitted until acceptance to allow anonymous review) Author declarations section (conflict of interest, ethics approval, data availability statement) Appendices (optional) Endnotes Most papers also contain figures and/or tables (with captions) that "float" outside the sequential order of the main text so they can be placed at the top or bottom of a final printed page. In your initially submitted manuscript, place each figure or table near where it is first referenced, without assuming that it will stay in that exact location when the paper is published. --> To allow two-way anonymous review, you should omit author name(s), affiliation(s), and acknowledgements from your initially submitted manuscript. (You will still provide this information to the editor, via the manuscript submission form.) If and when your manuscript is conditionally accepted, be sure to include name(s) and affiliation(s) in your editable manuscript file. Changes to citations may also be necessary, but the submitted paper should allow reviewers to access all necessary information; please read here for more details. You may choose to have your Chinese, Japanese, or Korean names published in your own language alongside the English versions in the author list. For further information, please see AIPP’s guidelines for Chinese, Japanese, or Korean names. Please refer to the sample manuscript pdf for the correct typographical and numbering conventions for each of these elements. LaTeX/REVTeX will take care of these conventions automatically. If you use MSWord, following the right conventions is your responsibility. -->
The abstract should summarize the paper’s contents as concisely as possible. It should make the goals of the paper clear and state the main results or conclusions directly. The abstract should be written so that any physicist, regardless of area of specialization, can read and understand it. Abstracts must be self-contained. They may not contain references to endnotes. Abstracts are optional in the Notes and Discussions section, but are encouraged for Notes longer than 1000 words.
Introduction
A paper's introductory section must provide the background and context that a typical physicist, regardless of area of specialization, would need in order to understand the paper's purpose and importance. That is, it should motivate the paper, in a way that is both informative and inviting. Unlike the abstract, the introduction need not summarize the entire paper or state its main results. Often, however, the introduction ends with a paragraph that outlines how the rest of the paper is organized; this is especially useful for longer papers.
When an equation is important, large, or complicated, display it on a line by itself, with a number (in parentheses) at the right margin. (In LaTeX, just use the equation environment.) Every equation, whether displayed or not, must be part of a complete sentence, with correct punctuation before and after. See the sample manuscript pdf for examples. All displayed equations should be numbered. When referring to an equation by number, put the number in parentheses and abbreviate "Eq." unless it is at the beginning of a sentence: "Equation (5) follows from substituting Eqs. (2) and (3) into Eq. (4)." While the copy editors will correct issues of style, such as putting letters in italics and distinguishing minus signs from hyphens, reviewers will appreciate more professional formatting. LaTeX math mode takes care of this typography automatically, but MSWord users will have to make a special effort. For all but the simplest expressions, MSWord users should use MathType or the built-in MSWord equation editor. Do not use any other equation editor, and be sure to use only standard fonts.
Please refer to our detailed instructions for figure preparation . Number figures in the order in which they are referred to in the text. Provide an appropriate and concise caption for each figure. When referring to a figure, abbreviate "Fig." unless it is at the beginning of a sentence: "Figure 5 shows the results of the new analysis in the same format as Fig. 4."
Number tables using Roman numerals, in the order in which they are referred to in the text. Provide an appropriate and concise caption for each table. Place each table as close as possible to the text that refers to it.
A concluding section is customary but not required. A good conclusion provides additional insights; it is not a summary. Summaries are not appropriate in AJP.
Supplementary Material
Authors can share additional material that is too long to fit in the published paper or may be interesting only to a subset of readers in the form of Supplementary Material. Examples of appropriate supplementary material include lengthy derivations, additional applications, large data tables, additional figures, computer programs, multimedia files, and curricular materials.
At initial submission, please upload your supplementary material either as a .zip file or as individual files. (The zip file is helpful for individual files whose format is not recognized by our manuscript system.) These materials should not contain any identifying information , although this can be added at acceptance. If it is difficult to remove personally identifiable information from the materials, please consult the editor in advance ( [email protected] ). In the body of your paper, you should use the phrase “supplementary material” to refer to these files, which will be available to reviewers.
AIP Publishing has partnered with Figshare—a general-purpose repository—to host all supplementary material for published papers. After your manuscript has been accepted for publication, AIP Publishing will deposit your supplementary material in Figshare on your behalf, where it will be assigned a digital object identifier (DOI) for citation and referencing. This increases the discoverability of your materials due to the searchable, user-friendly Figshare platform, which contains analytics and usage data to note the impact and influence of your work.
Additional information about Figshare can be found here .
Once your paper containing supplementary material has been conditionally accepted, please create a new section of your paper entitles Supplementary Material and place it after the main text and before the acknowledgements. Sample text for that section is: “Please click on this link to access the supplementary material, which includes the Mathematica code and also works out the application of this theory to three objects. Print readers can see the supplementary material at [DOI to be inserted by AIPP].” You would, of course, modify the phrase "includes the Mathematica code and also works out the application of this theory to three objects” to match what you have uploaded.
In the body of your paper, wherever you have used the phrase “supplementary material” to refer to this material, the phrase will become a hyperlink that will take online readers directly to the supplementary material section of the paper.
You will maintain the rights to this material and may choose to share it under any of these open access licenses: CC-BY 4.0, CC-BY-NC 4.0, or CC0.
Note that all supplementary material must be approved by the editor as part of the acceptance process. Your supplementary materials will be shared exactly as provided; no text editing or formatting is performed.
Acknowledgments
Acknowledgments should be omitted until a paper is conditionally accepted to allow anonymous review. When submitting the accepted paper, be sure to acknowledge colleagues who contributed in a significant way to your paper, as well as any funding agencies that supported your work. While it is not appropriate to acknowledge the assistance of the editors, it is often appropriate to acknowledge specific help and advice from our generous, conscientious, and anonymous reviewers. Examples of specific help are suggestions for references, pointing out significant errors, and suggesting better ways of doing calculations or experiments.
Author Declarations Section
All articles (but not editorials, book reviews, notes, letters, comments, and responses) should contain a Conflict of Interest statement and a Data Availability statement. Any paper that includes experiments using animals or humans needs to contain an ethics approval statement. More information on these statements can be found here .
Use appendices for material that is less interesting than the rest of the paper but still needed for completeness. Examples might include a technical proof, or a detailed description of research protocols. If there is more than one appendix, label them with capital letters A, B, and so on.
Endnotes and Citations
AJP papers should not include a comprehensive listing of all the papers that have been published on a topic, or even the most important ones. Instead, the references should be a carefully curated list of resources that will be most useful to readers. Citations should (1) recognize when an idea was first developed in another source—in that case, give only the 1st occurrence; (2) give readers a place to find essential background that can’t be provided in this paper; (3) provide interested readers with places to find specific additional information. The paper should make it clear to readers exactly why each reference is cited and what readers will find there. For long references, such as textbooks, direct readers to the most useful sections. A note for authors of Physics Education Research papers: This citation policy conflicts with normal practice in PER, where authors are careful to cite all related work. AJP's primary goal is to serve the reader, not the researcher, so citations should be kept to a minimum even for these papers. AJP does not use footnotes, which appear at the bottom of a page; instead, AJP uses endnotes. Endnotes may include auxiliary author information, literature citations, and explanatory annotations. --> AJP uses endnotes for auxiliary author information, literature citations, and explanatory annotations. Alternately, brief explanatory annotations can be included as footnotes. These can be indicated using standard symbols such as *, †, ‡, §, ¶, #. Endnotes must be grouped together at the end of the manuscript, in the same sequence in which they are first referenced in the body of the manuscript. Citing websites should be done with care. AJP is an archival journal, and readers in 50 years (or 1 year!) may be disappointed to find that the website no longer exists. For that reason, it is normally preferable to cite journals or textbooks that will be accessible indefinitely. On the other hand, websites are sometimes the best source of information (such as product descriptions), and they will be accessible to readers who have limited library support. Authors are encouraged to consult with the editor about the best sources to cite. To avoid ambiguity , place superscripts where they won’t be mistaken for mathematical exponents. Within the body of the manuscript, citations to endnotes should appear as superscripts placed after any punctuation. Copy editors will normally correct the placement of citations with respect to punctuation, but they will not normally move them to a different position in the sentence; it is the author’s responsibility to place them where they minimize interruption (normally at the ends of sentences). References can also appear as "online citations," for example, ". . . as shown by Eq. (5) in Ref. 3, . . . " Endnotes may refer to each other (usually using an online citation as above), but may not introduce any new endnotes. The abstract may not contain citations to endnotes. Authors who use bibtex or other reference software must incorporate the references within the LaTeX file. Consult online sources such as this one for assistance. Literature References Copy editors will correct the format of references in accepted papers. However, reviewers will appreciate having them formatted in the AJP style described below. Endnote references to articles in periodicals should have the following form:
Freeman J. Dyson, "Feynman's proof of the Maxwell equations," Am. J. Phys. 58 (3), 209–211 (1990).
Note that unlike many journals, AJP requires that each article reference include the article title and its ending(as well as beginning) page number. Use of the issue number is encouraged but not required unless the periodical is paginated by issue (for example, Physics Today). See the AIP Style Manual for a list of standard periodical abbreviations.
An endnote reference to a book should have the following form (include page number or numbers when appropriate):
David J. Griffiths, Introduction to Electrodynamics , 2nd Ed. (Prentice Hall, Englewood Cliffs, NJ, 1989), pp. 331–334.
Example of an article in an edited volume:
M. R. Flannery, “Elastic scattering,” in Atomic, Molecular, and Optical Physics Handbook, edited by G. W. F. Drake (AIP Press, New York, 1996), p. 520.
In all book and article references, pay special attention to the use and placement of punctuation. Note that article titles are in quotes, while book titles are in italics. List authors' names in the format "Bradley W. Carroll and Dale A. Ostlie" when there are two authors, or "Harvey Gould, Jan Tobochnik, and Wolfgang Christian" when there are three or more. If there are four or more authors you may use the form "William H. Press et al."
References to online material should include a brief description and/or title.
For a reference to material that has not been published in print or online, provide as much information as possible and include "(unpublished)" in the citation. See the AIP Style Manual for examples.
Authors are urged to consult recent articles published in AJP to find additional examples of correctly formatted references.
Authors of accepted papers are encouraged to prepare video abstracts. These two-minute videos can serve as attractive introductions to a paper. Interested readers can see more information and a complete list of video abstracts.. Online readers will see the video abstract on the manuscript page. If you wish to inform print-based readers that there is a video abstract, you can include a reference to it in the text. The citation could read, "Please see the video abstract for this paper at [URL to be inserted by AIPP] or the link from the online version of this article at https://aapt.scitation.org/journal/ajp."
Supplemental Material
Supplemental material allows authors to easily share additional material that is too long to fit in the published paper or may be interesting only to a subset of readers. Examples of appropriate supplemental material include lengthy derivations, additional applications, large data tables, additional figures, computer programs, multimedia files, and curricular materials. A url link will be included in the reference section of the published article to allow users to navigate directly to the associated files. Supplemental material can be uploaded, along with the manuscript file, as part of the submission or re-submission process. When the material is made available through the website as part of the published paper, the filenames will be unchanged from the uploaded files, so the choosing descriptive filenames before uploading is encouraged. If the file format is not supported by PXP, you can create a zip file that contains it. The presence of supplemental material should be noted in a citation in the text, such as, "See supplemental material for the Mathematica code, x " where "x" is the number of an endnote. If the supplemental material is included during the review process, then the endnote should say, “ x See supplemental material at the reviewer website.” Once the manuscript is conditionally accepted, then the endnote should be changed to, “ x The Mathematica code is available at [url to be inserted by AIPP],” or, if there are multiple files, then, for example, “ x Supplemental material is available at [url to be inserted by AIPP].” There is currently a 100 MB limit on uploads via the AJP submission page, so please consult the editor if you need to submit supplemental materials that are larger than this. All supplemental material is posted online exactly as provided by the author. AIP Publishing makes no changes to the supplemental material files, including text editing or file conversion. All supplemental material for publication must be approved by the Journal Editor as part of a manuscript's normal review process. The author retains full rights to the supplemental material.
Online Multimedia
For audio and video files, an alternative to supplemental material is to have the multimedia material linked to a figure in the online version of your article. To do this, create a figure with a caption, numbered in sequence with any other figures, for each multimedia file. The figure content should be a still image from the video, or any small, reasonable placeholder image for audio. Write the figure caption to say “enhanced online,” with the statement “[url will be inserted by AIPP].” Include the multimedia file in your file uploads, choosing the file category “Integral Multimedia (enhanced audio/visual version of figure).” Additional information can be found on the AIPP website . The above instructions apply to papers that have completed peer review. To allow reviewers to see audio or visual files, these files should initially be uploaded as supplemental material. They can be changed to online multimedia following successful peer review.
No products in the cart.
Mtg.in will be in maintenance mode from 7:45 AM to 9:00 AM on Wednesday, August 21, 2024. Please do not start the checkout after 7:40 am.

- Computer Science Olympiad (ICSO) Books
- English Olympiad (IEO) Books
- GK Olympiad (IGKO) Books
- Maths Olympiad (IMO) Books
- Science Olympiad (NSO) Books
- Olympiads Combo Packs
- SST Olympiad (ISSO) Books
- Hindi Olympiad (IHO) Books
- International Computer Science Olympiad (ICSO) Books
- Commerce Olympiad (ICO) Books
- NEET Champion
- NEET Previous Years Paper
- NEET Sample Paper
- NEET Crash Course
- Online Tests
- NEET Biology Books
- NEET Physics Books
- NEET Chemistry Books
- Assertion & Reason
- AIIMS Previous Years Paper
- GK & Aptitude
- JIPMER Previous Years Paper
- JEE Main Guide
- JEE PREVIOUS YEARS PAPER
- JEE Sample Papers
- JEE Crash Course
- JEE Champion
- NCERT Fingertips
- JEE Mathematics Books
- JEE Physics Books
- JEE Chemistry Books
- KCET Books – Karnataka CET
- KEAM Books – Kerala Engineering Exam
- WBJEE Books – West Bengal JEE
- BITSAT Exam Books
- MHT CET Books – Maharashtra CET
- Live Classes
- Recorded Classes
- Monthly Magazine – Single Issue
- Monthly Magazines – Subscription
- Monthly Magazine: Older Volumes
- Foundation Course Class 6
- Foundation Course Class 7
- Foundation Course Class 8
- Foundation Course Class 9
- Foundation Course Class 10
- NCERT Solutions Class 6
- NCERT Solutions Class 7
- NCERT Solutions Class 8
- NCERT Solutions Class 9
- NCERT Solutions Class 10
- NCERT Solutions Class 11
- NCERT Solutions Class 12
- CBSE Class 9 Books
- CBSE Class 10 Reference Books
- CBSE Class 10 Sample Paper Books
- CBSE Class 10 Previous Year Solved Paper Books
- CBSE Class 11 Books
- CBSE Reference Books for Class 12
- CBSE Previous Year Papers class 12 Books
- CBSE Class 12 Sample Paper Books
- Competitive Books
- Olympiad Books – Combo
- Govt. Exams
- 21 Science Crossword Puzzles
- 51 English Grammar Worksheets
- IGKO Online Test Programme
- Literature Books
- Vedic Mathematics
- Science Skill Development
- Mathematics Skill Development
- English Skill Development
- Summer Programme Combo
- Psychometric Online Test
- General ebooks
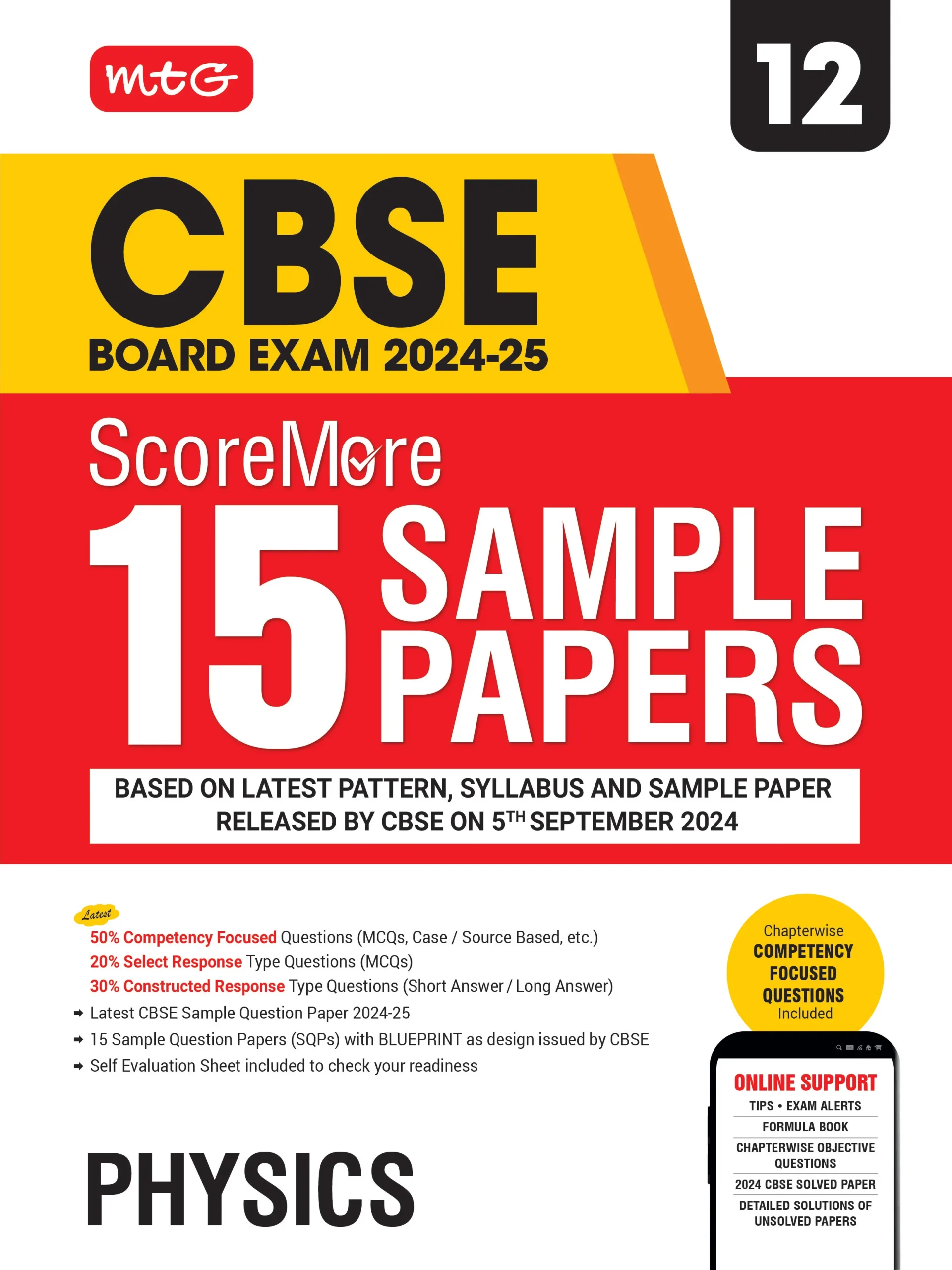
Click on the image to zoom in
Rating Rated 5.00 out of 5 based on 1 customer rating
Read reviews (1)
Write a review
CBSE ScoreMore 15 Sample Question Papers Class 12 Physics Book For 2025 Board Exam
₹ 275.00 Original price was: ₹275.00. ₹ 233.75 Current price is: ₹233.75. (15% Off)
Discount offer on this book in a bundle, click to view
Dispatch will start after 23rd September 2024
MTG has released the latest & updated CBSE ScoreMore 15 Sample Papers for the CBSE Session 2024-2025 of Class 12. It is most authentic and strictly based on the design of the latest sample paper and circular published by CBSE on 5 th September 2024. All the sample papers include all question typologies – Objective type and Subjective type. It is fully solved and adorned with self-evaluation sheets to check your readiness.
1000 in stock

Your security guaranteed
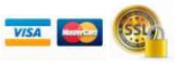
- Description
- Additional information
- Reviews (1)
MTG brought to you CBSE ScoreMore 15 Sample Papers for Class 12 aligned with the latest CBSE guidelines published on 5 th September 2024. It includes all types of question typologies on the current syllabus and pattern to help you practice more and score more in the CBSE Board exams in 2025.
Salient features of the book-
- 15 sample papers : 1 official CBSE sample question paper and 14 sample question papers according to the blueprint issued by CBSE .
- Each question paper covers all subjective and objective type questions based on brand new CBSE pattern –
- 50% Competency Focused Questions . (MCQs, Case/Source Based)
- 20% Select Response Type Questions . (MCQs)
- 30% Constructed Response Questions . (Short Answer & Long Answer Type Questions)
- Self-evaluation sheet is included to check your readiness and performance.
- Detailed solutions for 10 sample papers will be in the book & for 5 sample papers, it will be provided online to challenge your practice.
- Online support in the form of CBSE Tips, Exam Alerts, Formula Book, Learn & Retain, Chapter-wise Objective Questions, and 2024 CBSE Solved Paper.
ISBN13 | 9789360233112 |
---|---|
Author | MTG Editorial Board |
Edition | 2024-25 |
Pages | 224 |
Classes | Class 12 |
Exams | CBSE Boards |
Subjects | Physics |
Weight | 450gm |
1 review for CBSE ScoreMore 15 Sample Question Papers Class 12 Physics Book For 2025 Board Exam
utsabdutta97 – 11/25/2022
Easy to understand, Good content overall great book. Highly recommended for class 12 physics students 👍👍
Your email address will not be published. Required fields are marked *
Your review *
Name *
Email *
Save my name, email, and website in this browser for the next time I comment.
Customers prefer to buy this combination offer...

MTG CBSE ScoreMore 15 Sample Papers CBSE Boards -Class 12 Physics, Chemistry, Biology and English Combo
Total Price = ₹ 1,000.00
Combo Price = ₹ 800.00
Recommended for you
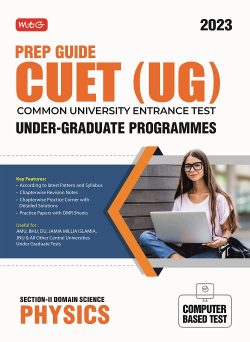
CUET (UG) PREP GUIDE SECTION:II PHYSICS 2023
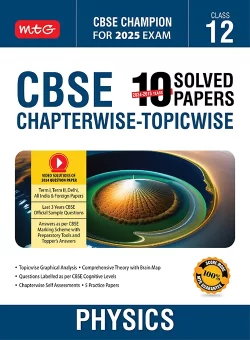
CBSE 10 Years Chapterwise Topicwise Solved Papers Class 12 Physics Book – CBSE Champion For 2024 Exam | CBSE Question Bank With Sample Papers (Based on Latest Pattern)
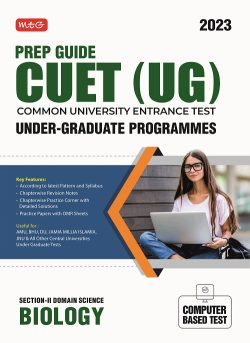
CUET (UG) PREP GUIDE SECTION:II BIOLOGY 2023
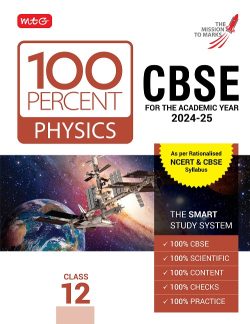
100 Percent Physics For Class 12 CBSE Board Exam 2024-25 | Chapter-Wise Self-evaluation Test, Theory, Diagrams & Practical Available All in One Book | As Per Rationalised NCERT & CBSE Syllabus
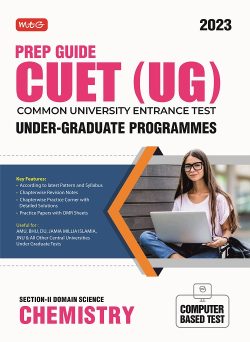
CUET (UG) PREP GUIDE SECTION:II CHEMISTRY 2023
Customers who bought this product also purchased....
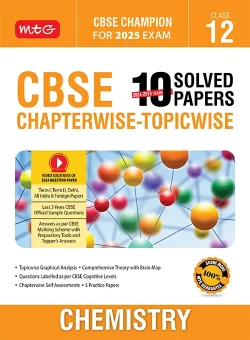
CBSE 10 Years (2024-2015) Chapterwise Topicwise Solved Papers Class 12 Chemistry Book – CBSE Champion For 2025 Exam | CBSE Question Bank With Sample Papers | Video Solution of PYQs (Based on Latest Pattern)
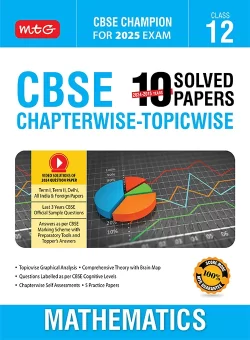
CBSE 10 Years Chapterwise Topicwise Solved Papers Class 12 Mathematics Book – CBSE Champion For 2024 Exam | CBSE Question Bank With Sample Papers (Based on Latest Pattern)
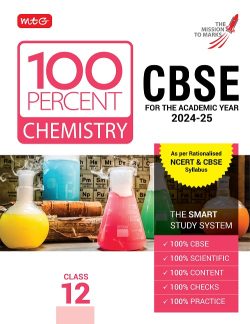
100 Percent Chemistry For Class 12 CBSE Board Exam 2024-25 | Chapter-Wise Self-evaluation Test, Theory, Diagrams & Practical Available All in One Book | As Per Rationalised NCERT & CBSE Syllabus
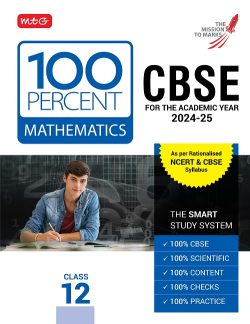
100 Percent Mathematics For Class 12 CBSE Board Exam 2024-25 | Chapter-Wise Self-evaluation Test, Theory, Diagrams & Practical Available All in One Book | As Per Rationalised NCERT & CBSE Syllabus
- Notifications
- Institutional Queries
Username or Email Address
Class Select Class Class 1 Class 2 Class 3 Class 4 Class 5 Class 6 Class 7 Class 8 Class 9 Class 10 Class 11 Class 12 Pre-Nursery
Phone number
Class Select Class Class 1 Class 2 Class 3 Class 4 Class 5 Class 6 Class 7 Class 8 Class 9 Class 10 Class 11 Class 12 Others Pre-Nursery
Country * Afghanistan Åland Islands Albania Algeria American Samoa Andorra Angola Anguilla Antarctica Antigua and Barbuda Argentina Armenia Aruba Australia Austria Azerbaijan Bahamas Bahrain Bangladesh Barbados Belarus Belau Belgium Belize Benin Bermuda Bhutan Bolivia Bonaire, Saint Eustatius and Saba Bosnia and Herzegovina Botswana Bouvet Island Brazil British Indian Ocean Territory Brunei Bulgaria Burkina Faso Burundi Cambodia Cameroon Canada Cape Verde Cayman Islands Central African Republic Chad Chile China Christmas Island Cocos (Keeling) Islands Colombia Comoros Congo (Brazzaville) Congo (Kinshasa) Cook Islands Costa Rica Croatia Cuba Curaçao Cyprus Czech Republic Denmark Djibouti Dominica Dominican Republic Ecuador Egypt El Salvador Equatorial Guinea Eritrea Estonia Eswatini Ethiopia Falkland Islands Faroe Islands Fiji Finland France French Guiana French Polynesia French Southern Territories Gabon Gambia Georgia Germany Ghana Gibraltar Greece Greenland Grenada Guadeloupe Guam Guatemala Guernsey Guinea Guinea-Bissau Guyana Haiti Heard Island and McDonald Islands Honduras Hong Kong Hungary Iceland India Indonesia Iran Iraq Ireland Isle of Man Israel Italy Ivory Coast Jamaica Japan Jersey Jordan Kazakhstan Kenya Kiribati Kuwait Kyrgyzstan Laos Latvia Lebanon Lesotho Liberia Libya Liechtenstein Lithuania Luxembourg Macao Madagascar Malawi Malaysia Maldives Mali Malta Marshall Islands Martinique Mauritania Mauritius Mayotte Mexico Micronesia Moldova Monaco Mongolia Montenegro Montserrat Morocco Mozambique Myanmar Namibia Nauru Nepal Netherlands New Caledonia New Zealand Nicaragua Niger Nigeria Niue Norfolk Island North Korea North Macedonia Northern Mariana Islands Norway Oman Pakistan Palestinian Territory Panama Papua New Guinea Paraguay Peru Philippines Pitcairn Poland Portugal Puerto Rico Qatar Reunion Romania Russia Rwanda São Tomé and Príncipe Saint Barthélemy Saint Helena Saint Kitts and Nevis Saint Lucia Saint Martin (Dutch part) Saint Martin (French part) Saint Pierre and Miquelon Saint Vincent and the Grenadines Samoa San Marino Saudi Arabia Senegal Serbia Seychelles Sierra Leone Singapore Slovakia Slovenia Solomon Islands Somalia South Africa South Georgia/Sandwich Islands South Korea South Sudan Spain Sri Lanka Sudan Suriname Svalbard and Jan Mayen Sweden Switzerland Syria Taiwan Tajikistan Tanzania Thailand Timor-Leste Togo Tokelau Tonga Trinidad and Tobago Tunisia Turkey Turkmenistan Turks and Caicos Islands Tuvalu Uganda Ukraine United Arab Emirates United Kingdom (UK) United States (US) United States (US) Minor Outlying Islands Uruguay Uzbekistan Vanuatu Vatican Venezuela Vietnam Virgin Islands (British) Virgin Islands (US) Wallis and Futuna Western Sahara Yemen Zambia Zimbabwe
Mobile number *
Email address *
Password *
Lost your password? Please enter your username or email address. You will receive a link to create a new password via email.
Username or email
Reset password
Just remembered? Log In Instead
Don’t have an account? Register with us

IMAGES
VIDEO
COMMENTS
。 SPM Physics Notes (Form 4, 5) : Modifications And Explanations (21) Sijil Pelajaran Malaysia. Calon SPM wajib menduduki semua kertas peperiksaan bagi mata pelajaran yang didaftar. Calon yang tidak dapat menduduki mana-mana kertas peperiksaan bertulis atau melaksanakan ujian lisan khusus calon persendirian akan dianggap TIDAK HADIR bagi mata pelajaran berkenaan. Calon yang tidak dapat ...
This is caused by the earth's gravitational force and air resistance.Using the appropriate physics concepts, suggest and explain suitable designs or ways to protect the satellite and its equipment: Suggestion Explanation Aerodynamic shape To reduce air resistance Heat shield / material that is hard to burn To protect the capsule High melting ...
SPM Physics KSSM physics form chapter force and motion explain why plasticine ball changes shape after dropped on block. how does front bumper and airbag helps. ... spring constant Get more extension Nylon fabric Not tear easily Net around trampoline Prevent children from slipping Modification for Pogo Stick Non slip material for handle.
Free essays, homework help, flashcards, research papers, book reports, term papers, history, science, politics ... physics spm essay example 2. ... Modification to be done to enable the electric bell to ring louder Increase the number Produced greater magnetic of turns of wire field to produce greater force. Increase the To increase the ...
This book contains all modifications and characteristics about SPM physics for Chapter 1 and 2 of Form 4: Force and Motion. For all Malaysian Students, who are taking SPM in Malaysia Form 4 and Form 5 Science Side, you can own this book to master the final question of Section B and C of your Physics exam Paper 2.
David Morin, [email protected] This chapter gives a brief introduction to quantum mechanics. Quantum mechanics can be thought of roughly as the study of physics on very small length scales, although there are also certain macroscopic systems it directly applies to. The descriptor \quantum" arises
FOR QUESTION ON MODIFICATION AND MAKING DICISION Chapter 1/2/3 (FORCES AND MOTION/PRESSURE) BIL ASPECT/CHARACTERISTIC/ MODIFICATION REASON/EXPLANATION 1 The small reading of scale 0.01 cm More sensitif/more accurate/suitable to measure a small length 2 Front and rear crumple zones to increase time of impact //to reduce impulsive force.
Impulsive Force. Impulsive force is defined as the rate of change of momentum in a reaction. Mathematically, we write F= mv−mu t. It is a force which acts on an object for a very short interval during a collision or explosion. A car of mass 1000kg is travelling with a velocity of 25 m/s. The car hits a street lamp and is stopped in0.05 seconds.
Physics Spm Paper 2 Tips 89 Modification and 11 Decision Making Question and Answer - Free download as PDF File (.pdf), Text File (.txt) or read online for free. The document provides suggestions and explanations for various designs and techniques. It discusses suitable designs for lab rulers, exercise equipment springs, high jump equipment, baby cradles, motorcycle shock absorbers, rockets ...
Feedback and control theory are important ideas that should form part of the education of a physicist but rarely do. This tutorial essay aims to give enough of the formal elements of control theory to satisfy the experimentalist designing or running a typical physics experiment and enough to satisfy the theorist wishing to understand its broader intellectual context. The level is generally ...
What is the function of the lead shot at the bottom of the hydrometer? A: To lower down the centre of gravity of the hydrometer. The hydrometer will topple if the centre gravity of the hydrometer is above the surface of the liquid. Forces Acted on Objects Immersed in Liquid. Archimedes Principle - Structure Question 1.
Speed increases. Wavelength increases. Wave refracted from normal. Frequency constant. Physics Tips SPM 2020 AG#1. 6 Diffraction of waves Spreading of waves after passing through a gap or a small obstacle. 6 Interference of Caused by superposition of waves from 2 coherent sources, resulting in.
The production of showers by the coalescence of cloud droplets. F. Ludlam. Physics, Environmental Science. 1951. The growth of unusually large cloud droplets by coalescence within isolated convective clouds is examined. It is found that if their initial radii are about 20 μ or more then growth into raindrops…. Expand.
Physics essays usually have a title, which sums up the essence of the investigation. It is based on the student's identified topic area. The research question further refines and defines the topic. It must be expressed as a question, not a statement. It must be expressed clearly and precisely, and appear early in the introduction of the essay
An electric motor converts electrical energy to kinetic energy. The diagram above shows the structure of a simple direct current motor (DC motor). It consists of a rectangular coil of wire placed between 2 permanent magnets. The coil is soldered to a copper split ring known as a commutator. 2 carbon brushes are held against the commutator.
This essay is a commentary on Physics I, with special reference to the account of change in chapter 7. It is argued: (i) that Aristotle is mistaken when he attempts in the earlier chapters to present his account as the natural development of various Pre-Socratic views 'on nature'; (ii) that as a result the main theme of his chapter 5 is a ...
19 Pages • Essays / Projects • Year Uploaded: 2021. This document discusses physics spm essay questions problem solving and decision making, in this document I include the hot essay questions from form 4 to form 5, very useful to student who is currently studying spm exam. This document is 15 Exchange Credits. Add to Cart.
A. Elements of good style for everyone. 1. Active and passive voices. You have heard this before, but it bears repeating: ac-tive sentences are more vigorous than passive ones. When you want to put more muscle into your prose style, re-place "Science is done by people" with "People do sci-ence.".
Experiment in Physics. Physics, and natural science in general, is a reasonable enterprise based on valid experimental evidence, criticism, and rational discussion. It provides us with knowledge of the physical world, and it is experiment that provides the evidence that grounds this knowledge. Experiment plays many roles in science.
Physics Essay Questions F4 Chapter 2 - Free download as Word Doc (.doc / .docx), PDF File (.pdf), Text File (.txt) or read online for free.
AJP papers should normally be 4000 to 6000 words, plus equations, tables, and figures if appropriate. As a rough rule, a double-spaced, 12-point manuscript of length x pages (including figures and equations) will require x/3 journal pages to print, and we aim for papers to be 6 pages or shorter. ... A note for authors of Physics Education ...
Physics Essays. We have physics writers ready and waiting to help. You might be looking for a physics essay that covers several themes like astrophysics and the exploration of astronomy, dealing with the physics of the universe; or a physics essay that looks at the vast array of biomechanics, including the study of the structure and function of biological systems such as humans, animals ...
This collection would be part or related to parts of the AI system I have described. Such an AI program would be very helpful to the research part of the narrowness problem but would probably not do much for the unity of physics. To maintain the breadth of physicists, projects like Physics or various evolutions of that journal are needed.
Dispatch will start after 23rd September 2024. MTG has released the latest & updated CBSE ScoreMore 15 Sample Papers for the CBSE Session 2024-2025 of Class 12.It is most authentic and strictly based on the design of the latest sample paper and circular published by CBSE on 5 th September 2024. All the sample papers include all question typologies - Objective type and Subjective type.